Translate this page into:
A simulation study to compare physiological responses to hypoxia and exercise between anaemic subjects and healthy controls
*Corresponding author: Dr (Prof). Biswajit Sinha, Department of Space and Environment Physiology, Institute of Aerospace Medicine (IAM)|Indian Air Force (IAF), Bengaluru, Karnataka, India. bsinha2001@yahoo.co.in
-
Received: ,
Accepted: ,
How to cite this article: Kumar A, Sinha B, Santhosh SR, Mishra P. A simulation study to compare physiological responses to hypoxia and exercise between anaemic subjects and healthy controls. Indian J Physiol Pharmacol 2022;66:181-7.
Abstract
Objectives:
Military flying is a complex task and requires high level of physical fitness of aviators. Various aviation stressors such as acceleratory force, hypoxia, cold weather conditions and decompression sickness place enormous stress on human physiological systems of the aviators. Individuals with Hb <13 g/dL (males) are being placed in the lower medical category which makes them unfit to fly. The present study was undertaken to assess the implication of low haemoglobin (Hb) on exercise capacity and hypoxia tolerance.
Materials and Methods:
Twenty-five individuals with anaemia and 15 healthy controls participated in the study. The participants were subjected to normobaric hypoxia (NH) equivalent to an altitude of 15,000 feet. Different physiological parameters such as heart rate (HR), systolic and diastolic blood pressure (SBP and DBP), respiratory rate (fR) and arterial oxygen saturation (SpO2) were measured during NH. Participants also performed maximal aerobic capacity (VO2 max) and maximal anaerobic capacity test in bicycle ergometer.
Results:
HR, SBP, DBP and fR in anaemic subjects were higher than healthy controls during NH, whereas SpO2 in anaemic subjects was lower as compared to healthy controls. Anaemic individuals had a lower VO2 max than their healthy counterparts. Critical power and anaerobic work capacity were lower in anaemic subjects than healthy controls.
Conclusion:
There is a deterioration in exercise capacity and hypoxia tolerance in individuals with low Hb levels. Adequate precaution should be exercised for permitting military aviators to fly with low Hb level.
Keywords
Anaemia
Hypoxia tolerance
Exercise tolerance
VO2 Max
Cardiovascular parameters
INTRODUCTION
High-altitude environment poses a number of serious challenges to human beings. This is characterised by low partial pressure of oxygen in the atmosphere which causes a reduction in partial pressure of oxygen in the blood known as hypoxia.[1] Hypoxia causes a deterioration in transport, storage and utilisation oxygen at the cellular level. Haemoglobin (Hb) carries 98% of oxygen transport in the blood in protein-bound form. In anaemia, when there is a reduction in Hb level either due to low number of RBCs or decrease in Hb concentration inside RBCs, oxygen-carrying capacity of the blood also reduces.[2]
Reduced oxygen transport associated with anaemia plays a crucial role in oxidative capacity of the cell. The reduced work productivity has been linked with reduced oxygen transport due to anaemia. Endurance capacity also gets compromised in moderate-to-severe iron deficiency anaemia.[3]
According to the existing guidelines, military aircrew with Hb <13 g/dL are being placed in lower medical category which makes them unfit to fly. It was hypothesised that anaemic individuals will have lower aerobic and anaerobic capacity and a lower tolerance to hypoxia. Physical work capacity and hypoxia tolerance are two important determinants of military flying. The present study was taken up to assess if individuals with borderline Hb levels may be permitted to fly based on their tolerance to hypoxia exposure and aerobic and anaerobic capacity.
MATERIALS AND METHODS
Twenty-five anaemic individuals were recruited in the present study. Anaemic individuals were again subdivided into three subgroups based on their Hb level, mild anaemic (12.0–12.9 g/ dL), moderate anaemic (11.0–11.9 g/dL) and severe anaemic (10.0–10.9 g/dL). Another 15 healthy participants with normal Hb level were taken as control subjects. The Institutional Ethics Committee approved the study protocol.
The following were the inclusion and exclusion criteria to select the participants in the anaemic group:
-
Inclusion criteria
The following criteria were included in the study:
Hb <13 g/dL
Male
Age: 20–40 years.
-
Exclusion criteria
The following criteria were excluded from the study:
Hb <10 g/dL
Beta-thalassemia cases
Cardiovascular abnormality
Respiratory abnormality
Any comorbid condition which affects exercise and any chronic disease.
The design of the present study was a randomised and case– control study.
The study was carried out in four sessions. In the first session, participants’ Hb was estimated. The second, third and fourth sessions were used for aerobic, anaerobic and hypoxia exposure evaluation, respectively. Hb estimation was done by Sysmex 3-part analyser.
Aerobic capacity of the participants was measured by standard dynamic cycle ergometry during maximal exercise test. Maximal oxygen consumption (VO2 max) was measured by cardiopulmonary exercise testing device, Quark CPET, COSMED, Italy. HR during maximal exercise was measured by polar Heart Rate (HR) monitor using a chest belt and receiver watch.
Anaerobic capacity of the individual was measured by standard anaerobic test method using a bicycle ergometer. Peak power generated and anaerobic capacity of the individuals were measured during anaerobic test.
Individuals were subjected to normobaric hypoxia in a Reduced Oxygen Breathing Device (ROBD) (hypoxicator) by administering 12.7% oxygen simulating an altitude of 15,000 feet. The exposure was continued for 30 min. Physiological parameters were recorded every 5 min during hypoxia exposure followed by post-exposure recovery 1 at 2nd min and recovery 2 at 4th min. HR was recorded by single-lead electrocardiography by placing three gel electrodes on anterior surface of the chest. Blood pressure (BP) was recorded by a non-invasive Electronic Sphygmomanometer. HR, BP and respiratory rate (RR) were measured in multi-para patient monitor, PETAS, KMA 900 (Turkey).
Determination of Critical Power (CP) and Anaerobic Work Capacity (AWC) was done in the laboratory using the technique described by Moritani et al.[4] Four dynamic work tests up to the onset of muscular fatigue were performed on the cycle ergometer in the maximum time or T limit beyond which the initial power output levels could no longer be maintained that is, when there was a drop in pedalling frequency below 30 rpm. This ergometer is a constant power device, so that such a drop in rpm results in torque increase that brings about a sharp and well-defined endpoint to an exercise bout. The power output levels were kept sufficiently high (200, 225, 250 and 275 watts) to lead to the onset of muscular fatigue. Rest period was provided between tests for a period of 1 h or longer if necessary, for HR to return within ± 5 bpm of the resting value. The total amount of work performed (W limit) was defined as the product of the imposed power loading P and the T limit (W limit = P.T limit). The relationship between W limit and T limit has been shown to be highly linear and can be described by the equation for a straight line
W Limit = a+ b (T limit)
CP and AWC were derived as slope coefficient (b) and Y intercept (a), respectively, of the line defined by the relationship between W limit and T limit. It has been suggested that CP represents the rate of energy supply, magnitude of which determines the maximal power at which a muscle can work without fatigue whereas AWC represents both the energy contained in the high-energy phosphorous compounds and that originating from the use of intramuscular glycogen and is not dependent on circulatory oxygen supply.
Data were analysed using a professional statistical package Statistica 8.0. Data were first checked for normality by Shapiro–Wilk ‘W’ Statistic. After ascertaining the normality, the data were analysed by parametric method. Descriptive statistical analysis of the parameters was carried out and the values were expressed as mean ± SD. Independent sample t-test was used to compare the data between anaemic individuals and healthy controls. The level of significance was set at P < 0.05.
RESULTS
Age, height and weight of the participants are shown in [Table 1]. Anaemic individuals were subdivided into three groups based on their Hb level. Mild, moderate and severe anaemic individuals had an Hb level of 12.0–12.9 g/dl, 11.0–11.9 g/dl and 10.0–10.9 g/dl, respectively.
Age | Height | Weight | |
---|---|---|---|
Healthy | 22.9±1.33** | 159.2±2.04 | 57.9±3.01** |
Anaemic | 29.0±3.62 | 160.4±3.15 | 63.0±2.98 |
Mild | 32.5±2.08 | 159±2.94 | 63.7±2.87 |
Moderate | 27.8±3.56 | 160.8±2.72 | 62.4±3.47 |
Severe | 29.0±3.46 | 160.3±3.87 | 63.3±2.44 |
Values are given as mean±SD. **P<0.01 as significantly different from anaemic groups
Body mass index, body surface area and Hb% in healthy and anaemic individuals are shown in [Table 2]. Healthy control had a lower body mass as compared to anaemic groups. As expected, healthy group had a significantly higher Hb concentration than anaemic groups.
BMI | BSA | Hb (%) | |
---|---|---|---|
Healthy | 22.8±1.13 ** | 1.7±0.04 | 14.9±0.91 *** |
Anaemic | 24.5±1.15 | 1.7±0.07 | 11.1±0.70 |
Mild | 25.2±1.43 | 1.75±0.06 | 12.2±0.14 |
Moderate | 24.1±0.97 | 1.72±0.06 | 11.3±0.23 |
Severe | 24.6±1.17 | 1.73±0.07 | 10.3±0.24 |
Values are given as mean±SD. **P<0.001 as significantly different from anaemic groups. BMI: Body mass index, BSA: Body surface area, Hb: Haemoglobin
Maximal aerobic exercise test data in healthy and anaemic individuals are shown in [Table 3]. Healthy individuals had a higher VO2 max, VCO2 max, RER max and a lower HR max, fR max and VE max than their anaemic counterparts.
VO2max | HR max | VEmax | fRmax | VCO2max | RER max | |
---|---|---|---|---|---|---|
Healthy | 58.4±5.42*** | 184.0±4.35*** | 60.9±2.46*** | 40.3±1.62*** | 3.9±0.19*** | 1.19±0.05** |
Anaemic | 45.0±2.53 | 191.6±3.02 | 70.3±1.89 | 55.9±7.58 | 2.9±0.15 | 1.16±0.02 |
Mild | 43.8±4.99 | 189.7±4.42 | 69.9±1.44 | 58.7±2.62 | 2.9±0.12 | 1.15±0.01 |
Moderate | 44.9±2.07 | 192.0±2.69 | 70.0±2.19 | 53.7±10.42 | 2.9±0.17 | 1.16±0.02 |
Severe | 45.2±1.82 | 191.8±2.86 | 70.8±1.70 | 57.5±2.56 | 2.9±0.16 | 1.16±0.01 |
Values are given as mean±SD. ***P<0.001 as significantly different from anaemic groups. VO2 max: Maximal oxygen uptake; HR max: Maximal heart rate; VE max: Maximal ventilation; fR max: Maximal breathing rate, VCO2 max: Maximal carbon dioxide output, RER max: Maximal respiratory exchange ratio
Anaerobic exercise test performance data in healthy and anaemic individuals are shown in [Table 4]. Healthy control had a significantly higher critical power (CP) and anaerobic work capacity as compared to anaemic group.
Critical power | Anaerobic work capacity | |
---|---|---|
Healthy | 180±70.25** | 620±114.94* |
Anaemic | 116.4±81.82 | 520.3±185.19 |
Mild | 73.2±32.28 | 430.5±83.42 |
Moderate | 132.2±88.77 | 538.5±171.55 |
Severe | 114.4±86.83 | 536±234.53 |
Values are given as mean±SD. *P<0.05; **P<0.01 as significantly different from anaemic groups.
[Table 5] is showing the arterial oxygen saturation (SpO2) and RR in healthy and anaemic individuals during exposure to normobaric hypoxia. SpO2 was significantly lower in anaemic individuals from controls at 15, 20, 25 and 30 min. Breathing rate was significantly higher in anaemic individuals from controls at 25–30 min and during recovery at 5 min.
SpO2 % (Mean±SD) | RR (Mean±SD) | |||
---|---|---|---|---|
Healthy | Anaemic | Healthy | Anaemic | |
Baseline | 98.6±0.50 | 98.6±0.50 | 11.0±1.03 | 11.2±0.96 |
5 min | 95.8±1.35 | 94.4±1.66 | 12.9±1.27 | 14.1±0.72 |
10 min | 93.1±2.38 | 90.1±2.55 | 15.1±1.06 | 16.6±1.44 |
15 min | 90.2±3.05 | 86.4±2.34* | 17.1±1.18 | 19.8±1.76 |
20 min | 88.3±3.77 | 81.0±2.72* | 19.5±1.35 | 22.7±1.94 |
25 min | 84.8±3.83 | 79.0±3.89* | 21.6±1.95 | 25.7±2.40* |
30 min | 81.0±4.07 | 75.2±5.58* | 23.5±1.55 | 28.8±2.99* |
Rec 1 | 89.4±3.11 | 88.7±5.02 | 17.3±3.17 | 22.8±3.10* |
Rec 2 | 93.1±3.31 | 92.3±3.92 | 15.0±2.37 | 18.2±2.70 |
Values are given as mean±SD. *P<0.05, significantly different from healthy controls. SpO2%: Arterial oxygen saturation in %; RR: Respiratory rate
HR in control and anaemic group during hypoxia exposure is shown in [Figure 1]. HR was significantly higher at baseline and during hypoxia exposure in anaemic group as compared to control group.

- Heart rate (bpm) in control and anaemic individuals during hypoxia exposure. BL: Baseline, Rec 1: Recovery 1 first 2 min of post-hypoxia exposure; Rec 2: Recovery 2 3-4 min of post-hypoxia exposure.
Systolic and diastolic blood pressure (SBP and DBP) during hypoxia exposure is shown in [Figures 2 and 3]. SBP and DBP increased significantly during hypoxia exposure. Furthermore, anaemic individuals had a higher SBP and DBP during hypoxia exposure as compared to controls.
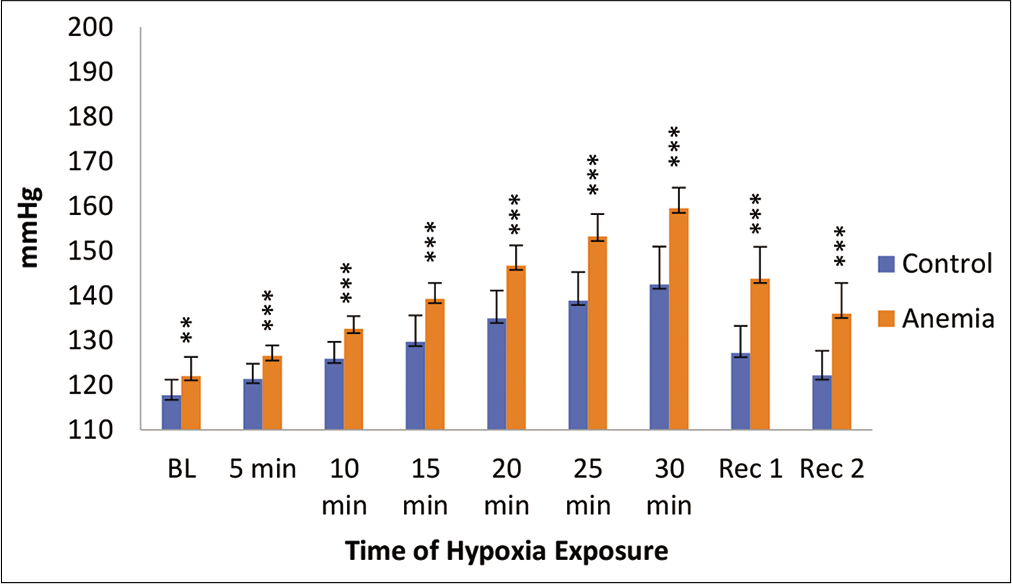
- Systolic blood pressure (mmHg) in control and anaemic individuals during hypoxia exposure. BL: Baseline, Rec 1: Recovery 1 first 2 min of post-hypoxia exposure; Rec 2: Recovery 2 3–4 min of post-hypoxia exposure.
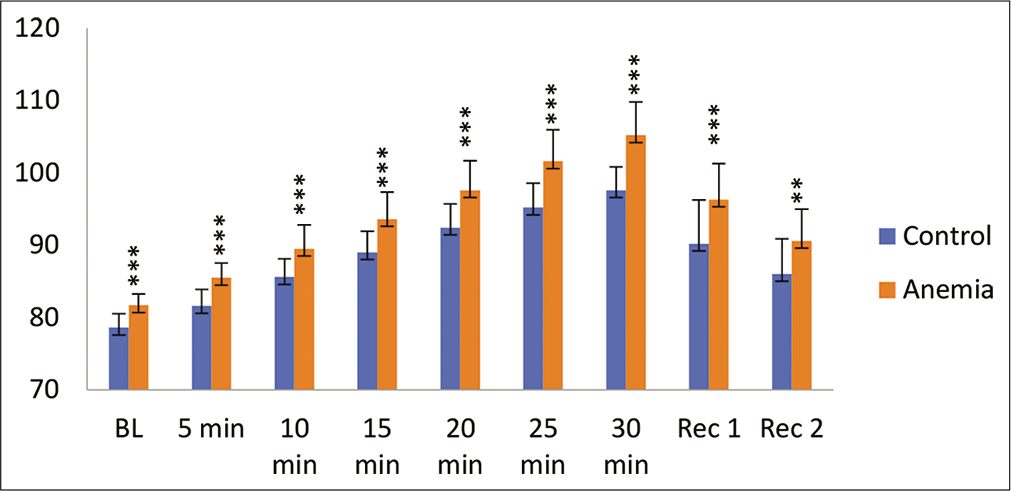
- Diastolic blood pressure (mmHg) in control and anaemic individuals during hypoxia exposure. BL: Baseline, Rec 1: Recovery 1 first 2 min of post-hypoxia exposure; Rec 2: Recovery 2 3–4 min of post-hypoxia exposure.
DISCUSSION
The present study examined the impact of low Hb level on exercise capacity and hypoxia tolerance in individuals with low Hb. Normobaric hypoxia was simulated using ROBD by administering 12.7% oxygen in the inhaled gas mixture for 30 min. Normobaric hypoxia was simulated equivalent to an altitude of 15,000 feet.
Anaemia has a negative impact on physical work capacity in different age groups as measured by changes in VO2 max and other cardiorespiratory parameters.[5-7] Anaemia is characterised by a decrease in the Hb levels or number of red blood cells, consequently leading to the oxygen-carrying capacity of red blood cells insufficient to meet the body’s oxygen demands. It has been reported in the literature that severe iron deficiency anaemia is responsible for a decline in work capacity and aerobic endurance exercise in particular.[8,9] VO2 max and CP were evaluated at different Hb levels in our study and it was found that there is no increasing pattern of VO2 max and CP when the Hb level increases.
Further, AWC was less in anaemic subjects compared to healthy controls. Anaemic individuals with very low Hb were not associated with very low anaerobic work capacity. This finding could be explained by that energy expenditure during anaerobic exercise is oxygen free, using intramuscular adenosine triphosphate (ATP) and creatinine phosphate as the major fuel and then lactate. Therefore, the effects of anaemia on oxygen carrying would not be so critical for anaerobic fitness.
CP, a measure of aerobic capacity, was found to be lower in anaemic individuals in the present study. VO2 max measurement provides an accurate and reliable information about the aerobic capacity of the individual. The anaemic individuals were found to have a lower VO2 max as compared to healthy control. The anaemic individuals were subdivided into three groups based on their Hb level. The three groups had an Hb level of 10–10.9, 11–11.9 and 12–12.9 g/dL, respectively. However, VO2 max did not differ significantly among different subgroups of anaemia.
Oxygen transport is a determining factor for anaerobic threshold in iron deficiency anaemia.[10] The lower the Hb level, the lesser is the anaerobic threshold. Anaerobic work capacity measured in CP-AWC model was found to be lower in anaemic individuals than their healthy counterparts. This reduction was not statistically significant, though. The literature had suggested that mild anaemia is not related to the lower anaerobic fitness. The energy expenditure for 2 min anaerobic exercises is oxygen free, using intramuscular ATP and creatinine phosphate as the major fuel in the initial 30 s and then lactate in the past 90 s.[11,12] On the other hand, a good correlation exists between aerobic fitness and mild anaemia.[13] The oxygen-carrying capacity of the blood reduces in anaemic individuals as Hb level reduces. The oxygenation of peripheral skeletal muscles is reduced, possibly impairing the exercise performance and thereby reducing the aerobic work capacity. As Hb is consumed by the pathological reasons to overtraining, aerobic fitness could be reduced as a result.[14]
The physiologic response to anaemia is a compensatory increase in cardiac output to maintain adequate oxygen delivery. Haemodynamic adaptations permit adequate cardiovascular compensation to occur in anaemic individuals.[15] The increased cardiac output is made possible by increases in blood volume, preload, HR and stroke volume, along with a decrease in afterload. Mean HR of anaemic subjects in our study during hypoxia exposure is more than the controls at all-time intervals including baseline value. Increased level of HR at baseline in anaemic subjects is due to compensation for the decrement in Hb level by an increase in blood flow. This may be accompanied by an elevated HR and/ or stroke volume. Resting HR in healthy individuals indicates the state of physical conditioning. The lower the resting HR, the better the physical conditioning. Anaemic individuals showed a higher resting HR than healthy controls.
The cardiovascular response to hypoxia is a dynamic process that commences immediately upon exposure. During acute hypoxia, sudden reductions in oxygen supply are compensated for by increases in oxygen delivery (cardiac output) which appear aimed at preserving or at least minimising reductions in conductive oxygen transport. The increase in cardiac output during acute hypoxia is due primarily to elevation in HR.[16] The balance between sympathetic and parasympathetic neural activity determines HR at rest and during acute exposure to hypoxia. The augmentation in sympathetic neural response during acute hypoxia exposure has been confirmed in many studies.[17,18] HR variability during acute hypoxia exposure has been used as a surrogate measure of autonomic nervous system function and has confirmed that a direct relationship exists between the magnitudes of hypoxia exposure and sympathetic activation.[19,20]
With acute exposure to normobaric or hypobaric hypoxia, the partial pressure of oxygen in the air, alveoli and blood is decreased. This results in oxyhaemoglobin desaturation and a reduction in arterial SpO2. To facilitate preserved tissue oxygen delivery, the cardiovascular system must upsurge systemic blood flow. Increase in HR during hypoxia exposure ensures optimum oxygen delivery to the central organs and peripheral tissues. The difference in HR between control and anaemic subjects at baseline (16 bpm) gradually reduces with the progression of hypoxia exposure. A difference of HR between healthy and anaemic individual of 15 bpm at 5th min decreases to 6 bpm at 30th min of hypoxia exposure. Findings of increased HR in anaemic subjects are similar to the study done by Gardner et al.,[7] Kapoor et al.[21] and Mani et al.[22]
SBP of anaemic subjects in our study during baseline and hypoxia exposure is higher than the healthy controls. Higher SBP signifies physical adaptations, namely increasing stroke volume and cardiac output. This finding is similar to the study done by Mani et al. and Kapoor et al. DBP of anaemic subjects in our study during baseline and during hypoxia exposure is higher than the healthy controls which can be explained on the basis of physical adaptations, namely stroke volume and cardiac output. These findings are similar to the study done by Kapoor et al.[21] and Alpert et al.[23] The resting SBP is significantly higher in anaemic children than control which is reported by Mani et al. They have also reported that recovery SBP after a treadmill test is slower in anaemic children.[22]
SpO2 of anaemic subjects in our study during baseline and during hypoxia exposure is lower in anaemic subjects. The blood Hb concentration is determinant of oxygen delivery. In anaemic patients, oxygen delivery decreases and oxygen extraction is increased. This leads to decreased venous Hb saturation and a lower tissue SpO2.
Exposure to a hypoxia environment, ventilation rises rapidly. During hypoxia exposure, the uptake of a given amount of oxygen requires a higher level of pulmonary ventilation than at sea level due to the lower partial pressure of oxygen. An increase in ventilation is one of the first adaptations to a hypoxic environment. This is known as hypoxic ventilatory response (HVR). HVR is initiated by stimulation of the peripheral chemoreceptors of the carotid bodies. HVR occurs in several phases, depending on the pattern, duration and intensity of the hypoxic stimulus.[24] Reduced oxygen level in the blood is sensed by the glomus cell of peripheral chemoreceptor.[25] The increase in RR during exposure to normobaric hypoxia is evident in healthy and anaemic subjects. However, the hypoxia stimulus causes intense activation of ventilatory response in anaemic subjects. In anaemic subjects, the binding of oxygen to Hb becomes compromised as Hb level is reduced. It may be conjectured that there is an intense activation of glomus cell in peripheral chemoreceptor leading to a comparatively higher ventilatory drive in anaemic individuals. The comparatively higher breathing rate is a compensatory mechanism to maintain the adequate oxygen level in the blood. During exercise, ventilatory drive becomes more in anaemic subjects as has been reflected in ventilation and breathing rate. When ventilatory response was equated with equivalent VO2 max between anaemic and control, it was observed that at a similar level of VO2 max, healthy control would have required a much lower ventilation to achieve similar VO2 in the blood. For example, healthy control had a VO2 max of 58.4 ml/min/kg and VE max of 60.9 L/min. For attaining a VO2 max of anaemic subjects that are 45.0 ml/min/kg, the healthy control would have required a ventilation of 47 L/min which is much lower than the ventilation max in anaemic subjects. By applying similar logic, when breathing rate was equated between two groups, it was found that the control group would have required a breathing rate of 30 breaths/ min for VO2 max of 45.0 ml/min/kg.
Anaemia causes decrease in aerobic work capacity. The main function of red blood cells in exercise is to transport oxygen from the lungs to the peripheral tissues and deliver metabolically produced carbon dioxide to the lungs for expiration. When the level of Hb drops, the oxygenation of extremity skeletal muscles is reduced, possibly impairing the exercise performance in advance. It has been mentioned in the study that severe iron deficiency anaemia is responsible for a decline in work capacity, particularly in aerobic endurance exercise.[8,9] The present study shows that there exists an association between anaemia and aerobic fitness. These findings prove that aerobic fitness is positively related to the overall capacity of Hb in red blood cells carrying oxygen in circulation to cardiac and skeletal muscles.
The effects of elevated altitude on the human body are numerous. In healthy individuals, HR increases at rest and at submaximal exercise workloads with no change in maximal heart rate. Increasing altitude causes a rise in SBP and decreased arterial SpO2[26] which is similar to the findings of the present study. Moschovis et al[27] reported that children with anemia pose a greater risk of developing cyanosis and a lower oxygen saturation at high altitude.
CONCLUSION
The present study reveals that unspecified mild anaemia is associated with the lower cardiorespiratory fitness and an accelerated ventilatory response during exposure to normobaric hypoxia. Hence, it is not desirable for military aviators to fly with low hemoglobin level.
Acknowledgement
The authors place on record a sincere thanks to the volunteers who agreed to participate in the study. The authors are grateful to the medical assistants of the department for their assistance in collecting the data. The Director General of Armed Forces Medical Services (DGAFMS) and Defence Research and Development Organization are thankfully acknowledged for sponsoring the project. Wg Cdr Amrita Parmar, Biochemist, is thankfully acknowledged for her contribution in submitting the proposal of the study.
Declaration of patient consent
The authors certify that they have obtained all appropriate patient consent.
Financial support and sponsorship
DGAFMS and DRDO.
Conflicts of interest
There are no conflicts of interest.
References
- High Altitude: Human Adaptation to Hypoxia. New York: Springer Publishers; 2014
- [CrossRef] [Google Scholar]
- Regulation of tissue oxygenation. Colloq Ser Integr Syst Physiol From Mol to Funct. 2011;3:1-100.
- [CrossRef] [Google Scholar]
- Iron deficiency and reduced work capacity: A critical review of the research to determine a causal relationship. J Nutr. 2001;131:676S-90.
- [CrossRef] [PubMed] [Google Scholar]
- Critical power as a measure of physical work capacity and anaerobic threshold. Ergonomics. 1981;24:339-50.
- [CrossRef] [PubMed] [Google Scholar]
- Prophylactic iron supplementation for underprivileged school boys III Impact on submaximal work capacity. Indian Pediatr. 1985;22:745-52.
- [Google Scholar]
- Cardiorespiratory, hematological and physical performance responses of anemic subjects to iron treatment. Am J Clin Nutr. 1975;28:982-8.
- [CrossRef] [PubMed] [Google Scholar]
- Hematological indices and iron status in athletes of various sports and performances. Med Sci Sports Exerc. 2002;34:869-75.
- [CrossRef] [PubMed] [Google Scholar]
- Incidence of iron deficiency and iron deficient anemia in elite runners and triathletes. Clin J Sport Med. 2017;27:493-8.
- [CrossRef] [PubMed] [Google Scholar]
- Effect of blood hemoglobin concentration on anaerobic threshold. Hokkaido Igaku Zasshi. 1991;66:458-67.
- [Google Scholar]
- The science and translation of lactate shuttle theory. Cell Metab. 2018;27:757-85.
- [CrossRef] [PubMed] [Google Scholar]
- Lactate kinetics at the lactate threshold in trained and untrained men. J Appl Physiol. 2013;114:1593-602.
- [CrossRef] [PubMed] [Google Scholar]
- Association between mild anemia and physical fitness in a military male cohort: The CHIEF study. Sci Rep. 2019;9:11165.
- [CrossRef] [PubMed] [Google Scholar]
- Prevalence and impact of anemia on basic trainees in the US air force. Sport Med Open. 2015;2:23.
- [CrossRef] [PubMed] [Google Scholar]
- Pulmonary gas exchange in humans exercising at sea level and simulated altitude. J Appl Physiol. 1986;61:260-70.
- [CrossRef] [PubMed] [Google Scholar]
- Arterial catecholamine responses during exercise with acute and chronic high-altitude exposure. Am J Physiol. 1991;261(4 Pt 1):E419-24.
- [CrossRef] [PubMed] [Google Scholar]
- Sympathetic response during 21 days at high altitude (4,300 m) as determined by urinary and arterial catecholamines. Metabolism. 1994;43:1226-32.
- [CrossRef] [Google Scholar]
- Effects of high altitude acclimatization on heart rate variability in resting humans. Eur J Appl Physiol Occup Physiol. 1996;73:521-8.
- [CrossRef] [PubMed] [Google Scholar]
- Augmented sympathetic activation during short-term hypoxia and high-altitude exposure in subjects susceptible to high-altitude pulmonary edema. Circulation. 1999;99:1713-8.
- [CrossRef] [PubMed] [Google Scholar]
- Cardiovascular responses to treadmill exercise testing in anemia. Indian Pediatr. 1997;34:607-12.
- [Google Scholar]
- Cardiovascular response in anemia. Indian J Pediatr. 2005;72:297-300.
- [CrossRef] [PubMed] [Google Scholar]
- Hemodynamic and ECG responses to exercise in children with sickle cell anemia. Am J Dis Child. 1981;135:362-6.
- [CrossRef] [PubMed] [Google Scholar]
- Control of breathing In: Swenson ER, Bärtsch P, eds. High Altitude Human Adaptation to hypoxia. New York, USA: Springer International Publishing; 2014. p. :36-55.
- [CrossRef] [Google Scholar]
- The patient with coronary heart disease at altitude: Observations during acute exposure to 3100 meters. J Wilderness Med. 1990;1:147-53.
- [CrossRef] [Google Scholar]
- Childhood anemia at high altitude: Risk factors for poor outcomes in severe pneumonia. Pediatrics. 2013;132:e1156-62.
- [CrossRef] [PubMed] [Google Scholar]