Translate this page into:
A randomised controlled trial to evaluate the use of genotyping and therapeutic drug monitoring versus only therapeutic drug monitoring as a strategy for risk minimisation in patients of epilepsy on phenytoin therapy
*Corresponding author: Mahesh N. Belhekar, Department of Clinical Pharmacology, Seth GS Medical College and KEM Hospital, Mumbai, Maharashtra, India. belhekardrmahesh4@gmail.com
-
Received: ,
Accepted: ,
How to cite this article: Belhekar MN, Vinayak A. A randomised controlled trial to evaluate the use of genotyping and therapeutic drug monitoring versus only therapeutic drug monitoring as a strategy for risk minimisation in patients of epilepsy on phenytoin therapy. Indian J Physiol Pharmacol. doi: 10.25259/IJPP_58_2024
Abstract
Objectives:
Phenytoin has a unique feature of accumulating in the body due to its non-linear elimination kinetics. Being a drug with a narrow therapeutic index, phenytoin needs routine therapeutic drug monitoring (TDM) to adjust the dose. Polymorphisms in cytochrome P450 (CYP [2C9 and C19]) genes reduce drug metabolism, increase drug concentrations and thus produce adverse drug reactions (ADRs). Detection of polymorphisms helps to predict the susceptibility to toxicity. Hence, this study was designed to compare the addition of genotyping to TDM of phenytoin as an investigational tool for assessing plasma levels and occurrence of ADRs in epileptic patients on phenytoin therapy as a part of risk minimisation.
Materials and Methods:
This randomised controlled trial was prospective and double-blind. Epileptic patients were randomised into two groups. One group received therapeutic doses of phenytoin based on their CYP2C9 and CYP2C19 genotyping, followed by monitoring drug levels by TDM. The other group was treated with phenytoin based on the clinician’s judgement. Monitoring of blood levels was done by TDM. Chi-squared test was used to analyse the difference in the occurrence of ADRs between the two groups.
Results:
In each group, Group A (genotyping and TDM) and Group B (TDM alone), 30 patients were enrolled, totalling 60 patients. Baseline characteristics of participants with or without CYP2C9 and CYP2C19 expression were statistically not significant. At the 6-month follow-up visit, in both CYP2C9 and CYP2C19 groups, the plasma concentration of phenytoin was statistically significant. We found 10 ADRs, and between the two groups, the difference in the occurrence of ADRs was non-significant.
Conclusion:
We found no significant utility of the addition of the genotyping in risk minimisation of phenytoin when used with TDM. Genotyping can be considered at the time of initiation of phenytoin therapy in epileptic patients.
Keywords
Adverse drug reactions
Genotyping
Phenytoin
Polymorphism
Risk minimisation
INTRODUCTION
Phenytoin is widely used as an anti-epileptic drug in all types of epilepsies other than absence seizures. One of its unique features is the risk of accumulation in the body as it is excreted by mixed-order kinetics of elimination. Being a potent enzyme inducer, it causes numerous drug-drug interactions and thus leads to the occurrence of many adverse reactions. Some of the adverse effects of chronic use of phenytoin at therapeutic plasma levels include acne formation, hirsutism, coarsening of facial features, vitamin deficiencies such as folate and vitamin D deficiency and gum hypertrophy. At toxic plasma levels, clinical features suggestive of CNS depression occur.[1] Hence, a proper risk management plan is necessary for phenytoin.
The risk management plan is defined as ‘a set of pharmacovigilance activities and interventions designed to identify, characterise, prevent or minimise risks relating to medicinal products including the assessment’.[2] One of the ways to prevent the harmful effects of drugs is through therapeutic drug monitoring (TDM), as it is helpful to rule out toxicity of drugs in contrast to manifestations of underlying disease processes for some drugs. TDM helps to speed up the drug treatment plan for every patient. Plasma levels of the drug class determined through TDM can guide the overall management of epilepsy.[3] The plasma concentration of phenytoin used for epilepsy is expected to be within the accepted laboratory reference range of 10–20 µg/mL.
In the case of anti-epileptic drugs (AEDs), even though plasma concentrations at therapeutic levels prevent or decrease the occurrence of seizures, it is always associated with side effects, but within an acceptable range.[4] TDM of AEDs, including phenytoin, has made it possible not only to identify variations in its utilisation but also to check for patient compliance and quality assurance aspects.[5] In addition, phenytoin, being a drug with a narrow therapeutic index, needs routine TDM to adjust doses.[6]
Genotyping is expected to be a promising tool to personalise phenytoin therapy, as mutations in several genes are thought to predispose patients to toxicity.[7-9] Phenytoin is a potent inducer of cytochrome P450 (CYP) enzymes, thus leading to drug interactions with drugs metabolised by these enzymes, such as immunosuppressants and oral anticoagulants.[10] Patient follow-up alone is not enough to foresee the occurrence of toxicity in a patient. As many drugs are metabolised and eliminated by CYP enzymes, which exhibit genetic polymorphism, their genotyping using pharmacogenetics is necessary.
Drug-specific guidelines for genotyping have been provided by the Clinical Pharmacogenetic Implementation Consortium (CPIC), based in the United States of America, which includes guidelines for drugs like phenytoin.[11] Genetic polymorphisms of the CYP2C subfamily exhibit remarkable inter-individual differences in the pharmacokinetics of phenytoin and will help us in predicting the high-risk group of epileptic patients on phenytoin therapy, who will be more prone to the development of ADRs. This will help to optimise the phenytoin dose for the individual patients and will help in minimising the occurrence of ADRs with the therapy. Ninety per cent of phenytoin metabolism occurs through an enzyme encoded by the gene CYP2C9 and 10% by the gene CYP2C19. Polymorphisms in these genes may decrease drug metabolism, causing increased plasma concentrations and leading to the occurrence of ADRs. Detection of these polymorphisms may help to predict the susceptibility to toxicity.[12]
As randomised clinical trials (RCTs) are often used to evaluate the efficacy of new intervention (here genotyping) against standard intervention (here TDM), we used RCT as a study design to compare the addition of genotyping to TDM of phenytoin as an investigational tool to assess the plasma levels of phenytoin as well as the occurrence of ADRs in epileptic patients on phenytoin therapy as a part of risk minimisation.
Rationale
It is difficult to predict clinically whether a patient is likely to suffer from phenytoin toxicity. Genotyping can be used as a potential tool to personalise phenytoin therapy and mutations in several genes which predispose patients to toxicity can be identified. TDM is routinely recommended to adjust doses. TDM helps to individualise drug therapy by analysing phenytoin levels and is helpful in decreasing the adverse events associated with phenytoin therapy. Genotyping can help in predicting the high-risk group of epileptic patients on phenytoin therapy who could be more prone to the development of ADRs. Then, we can optimise the phenytoin dose for the individual patients and will help in minimising the occurrence of ADRs with the therapy.
MATERIALS AND METHODS
The study was conducted as per the Indian Council of Medical Research guidelines 2017, and it was approved by the Institutional Ethics Committee (EC/OA-40/2019). It was registered with the Clinical Trial Registry of India (CTRI) (registration no - CTRI/2019/09/021317). This double-blind, randomised, controlled trial was conducted in two groups of epileptic patients on phenytoin therapy recruited from either the Neurology or TDM outpatient department of a tertiary care teaching hospital in India. The study period was 2 years, but it was extended due to the Coronavirus disease 2019 pandemic.
All patients aged 5–85 years, not exposed to phenytoin therapy in the last year (regardless of monotherapy or polytherapy), were included in the study. Patients with genotyping results (for CYP2C9 and CYP2C19 polymorphism) known due to prior testing or reports being available in the medical record, patients with a history of drug/alcohol abuse, patients with evidence of gastrointestinal tract, renal, endocrine, cardiovascular diseases, etc. and patients with status epilepticus were excluded from the study.
To the best of the investigators’ knowledge, during the planning of the study in the year 2019, there was no similar study conducted in an Indian setting to formally calculate a sample size. Hence, we decided to take a convenient sample of 30 patients in each group and a total sample size of 60 patients. After obtaining written informed consent/assent, the patients were randomised into two groups. One group (group A) received the therapeutic dose of phenytoin guided based on CPIC guidelines for dosing of phenytoin based on CYP2C9 genotyping, followed by monitoring of drug levels and modification of dose based on TDM levels. In another group (group B), genotyping was not performed initially, and dose administration was based on the clinician’s judgement and monitoring of the blood levels of phenytoin by TDM. Genotyping was done for this group of patients at the end of the study to compare the TDM levels between expression and non-expression of CYP2C9 and CYP2C19, as conducting genotyping anytime during the study period would introduce a bias for routine dosing in this group.
The randomisation plan was generated from http://www.randomization.com. As per this plan, the patients were divided into two blocks of randomisation with an allocation ratio of 1:1. Opaque, sealed envelopes were used as a method of allocation concealment.[13] Both patients and the observer (clinician) were blinded to the intervention received. The observer assessed only outcome measures. ADRs reported by the patients were recorded by the unblinded observer (clinician) during the scheduled follow-up visit, who also advised titration of the phenytoin doses in the lower range of recommended doses in case of occurrence of ADRs in any of the patients.
TDM of phenytoin in both groups was through estimation of trough levels of phenytoin concentrations. The participants were educated about the time gap to be maintained between the previous dose of phenytoin and the time of sampling. Patients were advised not to take the morning dose on the day of the visit, ensuring trough levels during sample collection. Under all aseptic precautions, four millilitres (mL) of venous blood were collected after completion of 12 h of the time of previous dosing. The morning dose was taken by the patient soon after the collection of the blood sample. The blood sample was centrifuged for separation of plasma, which was used for estimating trough plasma phenytoin levels using fast elution high-performance liquid chromatography by Chromaster, Japan. Quality control was maintained by running a three-level control sera provided along with the kits. These results of phenytoin TDM level concentrations performed prospectively for 1 year (1-, 3-, 6- and 12-month follow-up visits) in epileptic patients of either group were recorded in case record form. DNA extraction and, subsequently, genotyping were done using the remaining packed cells, which were stored at −80°C.
Genotyping studies were carried out using the cellular component by extracting the DNA and their amplification through polymerase chain reaction (PCR). PCR reactions were standardised for various parameters such as concentration of DNA, concentration of primers, deoxynucleotide triphosphates, magnesium chloride and annealing temperature. After optimising the reaction conditions, DNA samples of the subjects were amplified using primers specific to CYP2C9*2, CYP2C9*3, CYP2C19*2, CYP2C19*3 polymorphisms. The amplified product obtained was then subjected to enzyme digestion using a specific restriction enzyme. The product thus obtained was subjected to gel electrophoresis to identify the polymorphism.[14,15]
Statistical analysis
The baseline demographic data were summarised using descriptive statistics. Plasma levels of phenytoin and ADRs were recorded as categorical data and were summarised as frequency and percentages. The difference between the two groups regarding the occurrence of ADR was analysed using the Chi-squared test. All analyses were done at 5% significance.
RESULTS
Sixty patients with epilepsy on phenytoin therapy were enrolled in the study. The Consolidated Standards of Reporting Trials (CONSORT) flowchart is depicted in Figure 1.
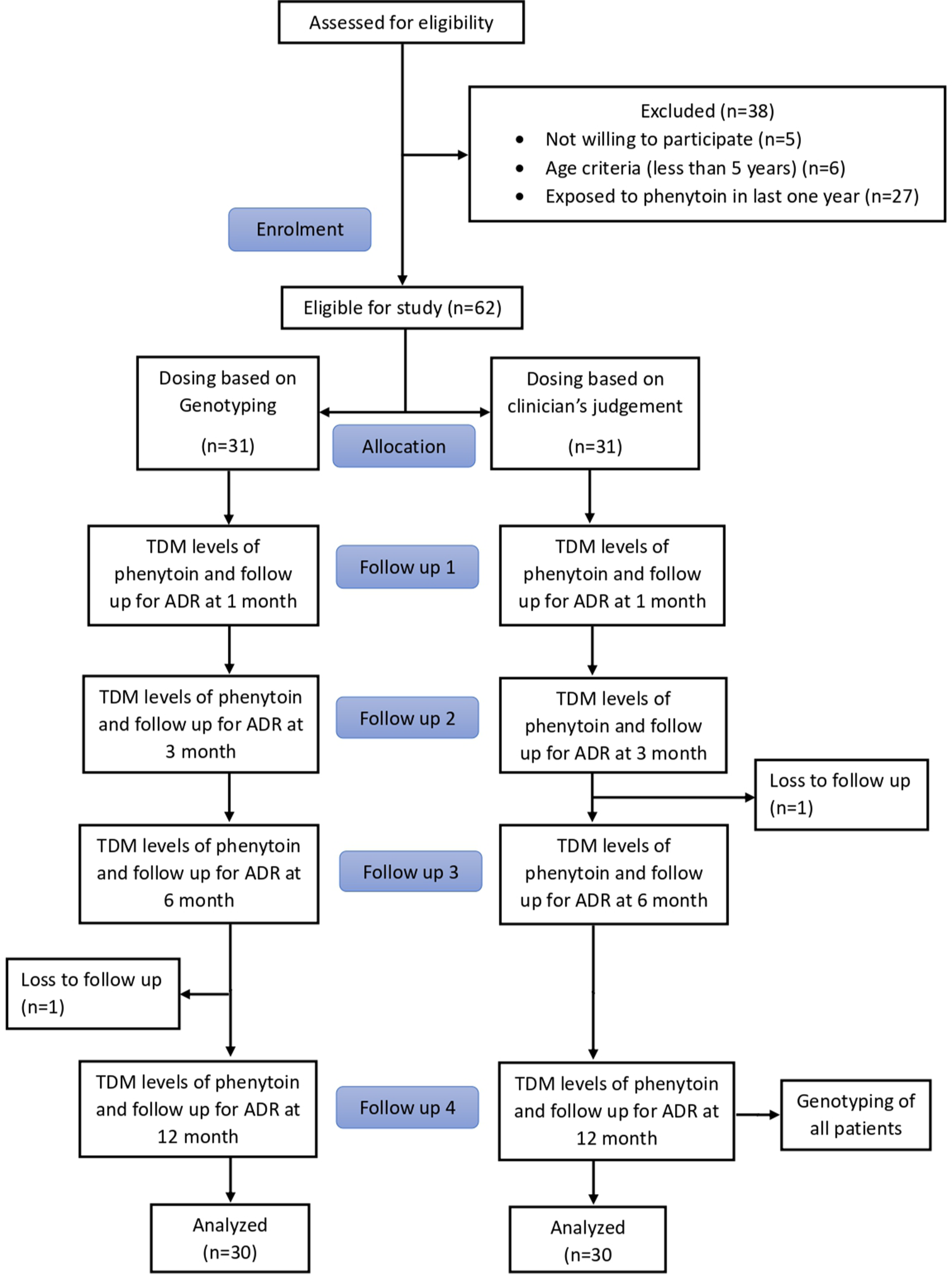
- CONSORT flowchart. TDM: Therapeutic Drug Monitoring, ADR: Adverse Drug Reactions
Demographic characteristics
Demographic measures of participants with or without CYP (2C9 and 2C19) expression are shown in Table 1.
Variable | Group A | Group B | Pvalue |
---|---|---|---|
Age (years) | 31.43±11.74 | 31.67±12.30 | 0.940$ |
Weight (kg) | 57.39±16.76 | 58.93±11.43 | 0.679$ |
Gender | |||
Female | 8 (26.67%) | 7 (23.33%) | 0.766# |
Male | 22 (73.33%) | 23 (76.67%) | |
Baseline plasma phenytoin levels (mcg/mL) | 0.676±0.899 | 0.889±1.443 | 0.496$ |
P-values calculated by $unpaired t-test and #Chi-squared test
The difference in the baseline characteristics was found to be statistically non-significant.
TDM levels over the entire follow-up period
Table 2 shows plasma phenytoin levels (μg/mL) over the entire study period.
Plasma phenytoin levels (mcg/mL) | Group A | Group B | Pvalue |
---|---|---|---|
1-month follow-up | 7.737±5.992 | 7.829±4.805 | 0.948 |
3-month follow-up | 8.947±3.998 | 8.522±2.769 | 0.634 |
6-month follow-up | 11.532±2.219 | 10.919±2.363 | 0.304 |
12-month follow-up | 11.830±2.273 | 11.021±2.438 | 0.189 |
P-values calculated by unpaired t-test. TDM: Therapeutic drug monitoring
Among the two groups (groups A and B), the difference in the plasma phenytoin levels was found to be statistically non-significant.
TDM levels in expressor and non-expressors
Plasma concentration of phenytoin (μg/mL) (TDM drug levels) at each visit of expressors and non-expressors of CYP2C9 and CYP2C19 is depicted in Tables 3a and 3b, respectively. The difference in TDM levels at 6-month follow-up visits was found to be statistically significant among CYP2C9 and CYP2C19 expressors and non-expressors and not at any other follow-up visit (1 month, 3 months and 12 months) in both groups.
CYP2C9 | |||
---|---|---|---|
Expressors (%) | Non expressors (%) | P -value | |
1-month phenytoin levels | |||
Below Normal | 39 (83) | 8 (17) | 0.357 |
Normal | 10 (90.9) | 1 (9.1) | |
More than Normal | 1 (50) | 1 (50) | |
3-month phenytoin levels | |||
Below Normal | 34 (85) | 6 (15) | 0.078 |
Normal | 16 (84.2) | 3 (15.8) | |
More than Normal | 0 (0) | 1 (100) | |
6-month phenytoin levels | |||
Below Normal | 7 (53.8) | 6 (46.2) | 0.004* |
Normal | 43 (91.5) | 4 (8.5) | |
12-month phenytoin levels | |||
Below normal | 6 (66.7) | 3 (33.3) | 0.163 |
Normal | 44 (86.3) | 7 (13.7) |
P-values calculated by Chi-squared test. * indicates statistically significant difference TDM: Therapeutic drug monitoring, CYP2C: Cytochrome P450 2C
CYP2C19 | ||||
---|---|---|---|---|
Expressors (%) | Non expressors (%) | P-value | ||
1-month phenytoin levels | ||||
Below Normal | 17 (36.2) | 30 (63.8) | 0.773 | |
Normal | 3 (27.3) | 8 (72.7) | ||
More than Normal | 1 (50) | 1 (50) | ||
3-month phenytoin levels | ||||
Below Normal | 15 (37.5) | 25 (62.5) | 0.273 | |
Normal | 5 (26.3) | 14 (73.7) | ||
More than Normal | 1 (100) | 0 (0) | ||
6-month phenytoin levels | ||||
Below Normal | 8 (61.5) | 5 (38.5) | 0.045* | |
Normal | 13 (27.7) | 34 (72.3) | ||
12-month phenytoin levels | ||||
Below Normal | 4 (44.4) | 5 (55.6) | 0.706 | |
Normal | 17 (33.3) | 34 (66.7) |
P-values calculated by Chi-squared test. * indicates statistically significant difference TDM: Therapeutic drug monitoring, CYP2C: Cytochrome P450 2C
Occurrence of ADRs in expressor and non-expressor groups
Table 4a depicts the number of participants with ADRs between the groups. There were ten ADRs in total. The differences in ADRs that occurred among the two groups (Group A and Group B) were not statistically significant.
ADR reported (%) | No ADR reported (%) | P-value | |
---|---|---|---|
Genotyping+TDM | 5 (16.67) | 25 (83.33) | 1 |
TDM alone | 5 (16.67) | 25 (83.33) |
P-values calculated by Chi-squared test. ADRs: Adverse drug reactions, TDM: Therapeutic drug monitoring
The occurrence of ADRs among expressors and nonexpressors of CYP2C9 and CYP2C19 is depicted in Tables 4b and 4c, respectively. The occurrence of ADRs was not statistically significant between the expressors and non-expressors.
CYP2C9 | |||
---|---|---|---|
Expressors (%) | Non expressors (%) | P-value | |
1-month follow-up ADR | |||
No | 46 (85.2) | 8 (14.8) | 0.259 |
Yes | 4 (66.7) | 2 (33.3) | |
3-month follow-up ADR | |||
No | 49 (84.5) | 9 (15.5) | 0.308 |
Yes | 1 (50) | 1 (50) | |
6-month follow-up ADR | |||
No | 49 (83.1) | 10 (16.9) | 1 |
Yes | 1 (100) | 0 (0) | |
12-month follow-up ADR | |||
No | 49 (83.1) | 10 (16.9) | 1 |
Yes | 1 (100) | 0 (0) |
P-values calculated by Chi-squared test. ADRs: Adverse drug reactions, CYP2C: Cytochrome P450 2C
CYP2C19 | |||
---|---|---|---|
Expressors (%) | Non expressors (%) | P -value | |
1-month follow-up ADR | |||
No | 20 (37) | 34 (63) | 0.412 |
Yes | 1 (16.7) | 5 (83.3) | |
3-month follow-up ADR | |||
No | 20 (34.5) | 38 (65.5) | 1 |
Yes | 1 (50) | 1 (50) | |
6-month follow-up ADR | |||
No | 21 (35.6) | 38 (64.4) | 1 |
Yes | 0 (0) | 1 (100) | |
12-month follow-up ADR | |||
No | 21 (35.6) | 38 (64.4) | 1 |
Yes | 0 (0) | 1 (100) |
P-values calculated by Chi-squared test. ADRs: Adverse drug reactions, CYP2C: Cytochrome P450 2C
DISCUSSION
Phenytoin was discovered in 1938 as an anticonvulsant, and since then, it has been used not only for the management of acute attacks of seizures but also for the prevention of the same.[16,17] In lower-middle-income countries like India, phenytoin is still used by a large population. For the treatment of partial and secondarily generalised seizures, even though phenytoin is replaced by valproate or carbamazepine, it is still preferred for the treatment of status epilepticus.[18,19] For drugs whose effects are difficult to measure clinically, as well as those with a narrow therapeutic index, the addition of genotyping to TDM is considered for the individualisation of the drug. Hence, this research was carried out in patients with epilepsy taking phenytoin therapy to find whether there is risk minimisation when genotyping is used along with TDM.
In this study, the average age of the study participants was 31.5 years with male preponderance. Our study findings concerning the average age and sex ratio of the participants were consistent with a study conducted in the same setting by Thakkar et al. (2012)[20] in the same setting. However, our findings are contradictory to the findings of Beghi and Giussani[21] which found that epilepsy has a bimodal distribution with high incidence in paediatrics and the elderly age group.
Phenytoin is a drug with a narrow therapeutic index and has a laboratory reference range of 10–20 µg/mL. In patients on phenytoin therapy, it is usually not easy to attain drug concentration in the recommended laboratory reference range due to wide inter-individual differences in the plasma concentrations achieved as well as the response seen from the recommended dose.[22,23] TDM is useful in enhancing therapeutic gains and reducing the occurrence of ADRs in patients on treatment with phenytoin, a drug with a narrow therapeutic index.[24] In this study, the mean baseline phenytoin plasma levels at the time of the patient enrolment were (0.783 μg/mL,) well below the laboratory reference range of (10–20 μg/mL), suggesting that the patients had either received no treatment or had been started on phenytoin therapy recently. This finding satisfied the inclusion criteria of the study. We found that the mean plasma phenytoin levels attained values within the normal range at the third follow-up visit (at 6 months) after starting therapy in both groups. This may be because phenytoin is 90–92% protein-bound and exhibits the phenomenon of autoinduction of its metabolising enzymes. Hence, there is a lag time for the attainment of stable target plasma levels.[9]
Pharmacogenetics can be used to minimise ADRs and to improve therapies, taking into account the variations in either pharmacokinetics or pharmacodynamics,[25] both of which are genetically encoded.[26] CYP genes encode many enzymes regulating both pharmacokinetics and pharmacodynamics.[27] The metabolism of phenytoin occurs primarily by the enzymes CYP enzymes (2C9-90% and 2C19-10%), and its plasma levels are influenced by their polymorphisms. However, several other factors (age, sex, dose, formulation, drug interactions and hepatic dysfunction) can influence plasma levels.[28]
In this RCT, at the 6-month follow-up period, we found a statistically meaningful distinction in the plasma phenytoin levels between CYP2C9 expressors and CYP2C19 nonexpressors. Among the CYP2C9 expressors, (43 out of 47 [91.5%]) patients had normal phenytoin levels, and among the CYP2C19 non-expressors, (34 out of 47 [72.3%]) patients had normal phenytoin levels. This suggests that the normal functioning of the CYP2C9 enzyme is one of the prime prerequisites for the maintenance of plasma phenytoin levels, as this enzyme metabolises the majority of it. However, the remaining phenytoin is metabolised by CYP2C19, and its decreased functioning does not alter the plasma levels.
In this study, ADRs occurred in 9 out of 10 [90%] patients who were CYP2C9 expressors and 10 out of 10 patients [100%] patients who were CYP2C19 non-expression, thus reemphasising the roles of the respective enzymes in phenytoin metabolism. The ADRs reported in this study consisted of non-serious ADRs listed in the FDA label, namely giddiness (n = 6), diplopia (n = 2), gingival hyperplasia (n = 1) and one case with symptoms of toxicity (ataxia, nystagmus, tremors and dysarthria). Among these, patients with plasma phenytoin levels below the laboratory reference range were 30%, and the patients with plasma phenytoin levels above the reference range were 20%. This is in contrast with the study conducted by Taur et al.[29] where 59% and 7% of the patients with ADRs, respectively, had plasma phenytoin levels above and below the reference range.
We have conducted this RCT as a major step forward in aligning TDM and pharmacogenetics with the factors affecting phenytoin risk minimisation. However, despite the study discovery, other hurdles bar the evolution and execution of pharmacogenetic tests, which include political, commercial, economic and academic barriers. These hinder the transmission of clinically useful information from the laboratory to the doctors as well as the patients. Hence, future studies need to focus on overcoming these hurdles.
Limitations
Small sample size and single-centred study.
CONCLUSION
It is concluded that there is no significant utility of the addition of the genotyping in risk minimisation of phenytoin when used with TDM. The PCR-restriction fragment length polymorphism technology used for the genotyping of phenytoin (as CYP2C9 and CYP2C19 genotypes – expressors or non-expressors) can be considered at the time of initiation of phenytoin therapy in epileptic patients and eventually, it will be used as a part of routine patient care in a public sector tertiary care hospital in India.
Acknowledgements
We thank the Head of the Department and Dean of our institute for their support and guidance.
Ethical approval
The research/study was approved by the Institutional Ethics Committee (IEC) at Seth GS Medical College and KEM Hospital Parel Mumbai, with project number EC/OA-40/2019, dated July 10, 2019.
Declaration of patient consent
The authors certify that they have obtained all appropriate patient consent.
Conflicts of interest
There are no conflicts of interest.
Use of artificial intelligence (AI)-assisted technology for manuscript preparation:
The authors confirm that there was no use of artificial intelligence (AI)-assisted technology for assisting in the writing or editing of the manuscript and no images were manipulated using AI.
Financial support and sponsorship: We are thankful to the following societies of our institute for sanctioning the intramural financial grants, for the conduct of the study: (i) Diamond Jubilee Society Trust (DJST) (grant number D1D35 dated 31 August 2020) (ii) Research Society (Research grants for 2019 - second set, dated 22 October 2019)
References
- Mechanisms of action of currently prescribed and newly developed antiepileptic drugs. Epilepsia. 1994;35(Suppl 4):S41-50.
- [CrossRef] [PubMed] [Google Scholar]
- Cost-effectiveness of therapeutic drug monitoring: A systemic review. Ther Drug Monit. 2005;27:10-7.
- [CrossRef] [PubMed] [Google Scholar]
- Test performance characteristics of the serum phenytoin concentration (SPC): The relationship between SPC and patient response. The Veterans Administration Epilepsy Cooperative Study Group. Ther Drug Monit. 1991;13:318-24.
- [CrossRef] [PubMed] [Google Scholar]
- Value of therapeutic drug monitoring in epilepsy. Expert Rev Neurother. 2008;8:929-39.
- [CrossRef] [PubMed] [Google Scholar]
- Clinical decision support of therapeutic drug monitoring of phenytoin: Measured versus adjusted phenytoin plasma concentrations. BMC Med Inform Decis Mak. 2012;12:7.
- [CrossRef] [PubMed] [Google Scholar]
- Clinical pharmacogenetics and potential application in personalized medicine. Curr Drug Metab. 2008;9:738-84.
- [CrossRef] [PubMed] [Google Scholar]
- Development of rapid genotyping methods for single nucleotide polymorphisms of cytochrome P450 2C9 (CYP2C9) and cytochrome P450 2C19 (CYP2C19) and their clinical application in pediatric patients with epilepsy. Yakugaku Zasshi. 2011;131:809-15.
- [CrossRef] [PubMed] [Google Scholar]
- The predictive value of MDR1, CYP2C9, and CYP2C19 polymorphisms for phenytoin plasma levels. Pharmacogenomics J. 2001;1:204-10.
- [CrossRef] [PubMed] [Google Scholar]
- Pharmacokinetic drug interactions with phenytoin (Part I) Clin Pharmacokinet. 1990;18:37-60.
- [CrossRef] [Google Scholar]
- Pharmacogenetics: A general review on progress to date. Br Med Bull. 2017;124:65-79.
- [CrossRef] [PubMed] [Google Scholar]
- Clinical pharmacogenetics implementation consortium guidelines for CYP2C9 and HLA-B genotypes and phenytoin dosing. Clin Pharmacol Ther. 2014;96:542-8.
- [CrossRef] [PubMed] [Google Scholar]
- Available from: https://www.sealedenvelope.com/simple-randomiser/v1/new [Last accessed on 2024 Feb 07]
- Allele and genotype frequency of CYP2C19 in a Tamilian population. Br J Clin Pharmacol. 2003;56:331-3.
- [CrossRef] [PubMed] [Google Scholar]
- The role of the CYP2C9-Leu359 allelic variant in the tolbutamide polymorphism. Pharmacogenetics. 1996;6:341-9.
- [CrossRef] [PubMed] [Google Scholar]
- Landmark article Sept 17, 1938: Sodium diphenyl hydantoinate in the treatment of convulsive disorders. JAMA. 1984;251:1062-7.
- [CrossRef] [PubMed] [Google Scholar]
- Evidence-based guideline: Treatment of convulsive status epilepticus in children and adults: Report of the guideline committee of the American Epilepsy Society. Epilepsy Curr. 2016;16:48-61.
- [CrossRef] [PubMed] [Google Scholar]
- Antiepileptic drugs prescription utilization behavior and direct costs of treatment in a national hospital of India. Ann Indian Acad Neurol. 2012;15:289-93.
- [CrossRef] [PubMed] [Google Scholar]
- Phenytoin versus valproate monotherapy for partial onset seizures and generalized onset tonic-clonic seizures. Cochrane Database Syst Rev. 2016;2016(4):CD001769.
- [CrossRef] [Google Scholar]
- Association of CYP2C9 polymorphisms with phenytoin toxicity in Indian patients. Neurol India. 2012;60:577-80.
- [CrossRef] [PubMed] [Google Scholar]
- Aging and the epidemiology of epilepsy. Neuroepidemiology. 2018;51:216-23.
- [CrossRef] [PubMed] [Google Scholar]
- Applied pharmacokinetics and pharmacodynamics principles of therapeutic drug monitoring. Am J Pharm Educ. 2006;70:148.
- [CrossRef] [Google Scholar]
- The serum level approach to individualization of drug dosage. Eur J Clin Pharmacol. 1975;9:1-8.
- [CrossRef] [PubMed] [Google Scholar]
- Overview of therapeutic drug monitoring. Korean J Intern Med. 2009;24:1-10.
- [CrossRef] [PubMed] [Google Scholar]
- Identifying genetic risk factors for serious adverse drug reactions: Current progress and challenges. Nat Rev Drug Discov. 2007;6:904-16.
- [CrossRef] [PubMed] [Google Scholar]
- Genetic susceptibility in pharmacodynamic and pharmacokinetic pathways underlying drug-induced arrhythmia and sudden unexplained deaths. Forensic Sci Int Genet. 2019;42:203-12.
- [CrossRef] [PubMed] [Google Scholar]
- Pharmacokineticpharmacodynamic consequences and clinical relevance of cytochrome P450 3A4 inhibition. Clin Pharmacokinet. 2000;38:41-57.
- [CrossRef] [PubMed] [Google Scholar]
- Clinical pharmacogenetics implementation consortium (CPIC) guideline for CYP2C9 and HLA-B genotypes and phenytoin dosing: 2020 update. Clin Pharmacol Ther. 2021;109:302-9.
- [CrossRef] [PubMed] [Google Scholar]
- An audit of therapeutic drug monitoring services of anticonvulsants at a tertiary care hospital in India. Ther Drug Monit. 2013;35:183-7.
- [CrossRef] [PubMed] [Google Scholar]