Translate this page into:
Cardiorespiratory responses to incremental exercise in dominant somatotypes
*Corresponding author: Geetanjali Gokarn Bade,b Department of Physiology, All India Institute of Medical Sciences, New Delhi, India. geetanjalibade@gmail.com
-
Received: ,
Accepted: ,
How to cite this article: Jaiswal K, Talwar A, Bade GG. Cardiorespiratory responses to incremental exercise in dominant somatotypes. Indian J Physiol Pharmacol. doi: 10.25259/IJPP_553_2023
Abstract
Objectives:
Somatotype rating is used to categorise human physiques using parameters related to body shape and composition. These parameters are adiposity, musculoskeletal robustness, and linearity. Somatotype rating is based on anthropometric dimensions that influence a person’s ability to perform physical activity. This study aimed to measure cardiorespiratory responses to incremental exercise in different somatotypes.
Materials and Methods:
Fifty (50) healthy male participants with a mean age of 24.10 ± 4.55 years were recruited in this study. The dominant somatotype was determined using the Heath and Carter method. Cardiopulmonary exercise testing was done to measure peak VO2, peak VO2/kg, metabolic equivalents (METS), breathing reserve (BR), minute ventilation (V.E), oxygen pulse (VO2/HR), and heart rate.
Results:
The cardiorespiratory parameters showed significant differences between the endomorphs and mesomorphs. Mesomorphs showed significantly higher peak oxygen consumption (mL/min) (2487 ± 364.3 vs. 2151 ± 287.8; P = 0.013) and exhibited significantly higher peak VO2 % predicted values (78.13 ± 10.11 vs. 66.92 ± 10.09; P = 0.003) than the endomorphs. METS % predicted was significantly higher in mesomorphs than endomorphs (78.13 ± 11.95 vs. 66.92 ± 10.09; P = 0.003). Similarly, the V.E (L) of mesomorphs was also higher than that of endomorphs (95.32 ± 18.63 vs 71.13 ± 25.39), but the BR (L) of mesomorphs was lower than endomorphs (38.38 ± 11.87 vs 53.80 ± 17.16) and ectomorphs (38.38 ± 11.87 vs 52.79 ± 9.47). Mesomorphs showed higher O2 pulse than endomorphs (14.8 [10.3–24.0] vs 12.8 [9.2–21.9]; P = 0.02); they also showed significantly higher respiratory frequency than endomorphs (1/s) (48.25 ± 11.34 vs. 38.19 ± 6.89; P = 0.001) and ectomorphs (48.25 ± 11.34 vs. 40.92 ± 7.03; P = 0.03).
Conclusion:
Cardiorespiratory responses to exercise vary among different somatotypes. Exercise capacity, as measured by VO2 peak, is higher in mesomorphs.
Keywords
Cardiopulmonary exercise test
Peak oxygen uptake
Somatotypes
INTRODUCTION
Somatotype rating is used to categorise human physiques using parameters related to body shape and composition. These parameters are adiposity, musculoskeletal robustness and linearity or slenderness. W.H. Sheldon first used the word “somatotype” in 1940.[1] The word ‘somatotyping’ today refers to a variety of method-specific procedures, all of which are based on the original Sheldonian notions and use a three-component rating system. Heath and Carter pioneered the most frequently utilised somatotyping technique. This technique has three components – endomorphy, mesomorphy and ectomorphy – that empirically characterise relative fatness, relative musculoskeletal development and relative body linearity, respectively.[2] For example, a 2-5-1 rating expressed in this form would read as two for endomorphy, five for mesomorphy and one for ectomorphy. Thus, these figures indicate the magnitudes of the three components. A rating of ½–2½ for a component is considered low, 3–5 is considered moderate, 5½–7 is high, and 7½ and above is very high.[3]
It is known that anthropometric parameters influence the ability to perform physical activity. Heath and Carter’s anthropometric method of somatotype determination takes into consideration ten anthropometric parameters. These are height, weight, skinfold thickness (triceps, subscapular, supraspinal and medial calf), bone breadth (bi-epicondylar humerus and femur) and limb girth (arm flexed and tensed calf). It is a better predictor of body composition than other methods.[4] Compared to only using body mass index (BMI) and waist-hip ratio, this technique provides more accurate data on body composition. Thus, somatotype is a crucial component of someone’s physical fitness and health profile. During physical activity and training, people of various somatotypes exhibit unique performance capabilities.[5] It has been demonstrated that the dominant somatotype affects functional responses during maximal exercise.[5,6]
Cardiopulmonary exercise testing (CPET) offers a comprehensive evaluation of integrative exercise responses encompassing the pulmonary, cardiovascular, hematopoietic, neuropsychological and skeletal muscle systems that are not adequately reflected by the measurement of individual organ system function.[7] Hence, the objective of the present study was to measure cardiorespiratory responses to incremental exercise in different somatotypes.
MATERIALS AND METHODS
This study was approved by the Institute Ethics Committee for Human Subjects (Ref. No.: IECPG-520/23.09.2021, RT-02/28.10.2021 dated 29.10.21).
Written informed consent was taken from all participants as per the institute’s ethical norms.
Participants
This is an observational and cross-sectional study. After screening 75 healthy volunteers, 50 participants were enrolled in the study. A detailed history was recorded, and a physical examination was conducted to rule out the presence of any disease. Healthy volunteers who were willing to participate in the study were enrolled based on the following inclusion and exclusion criteria – the young (age group 18–35 years) healthy male (as exercise capacity is affected by gender) participants having only dominant somatotypes were recruited. Somatotype dominance is defined as a component rating for one somatotype that is at least 0.5 points greater than the rating of the other two components (for example, 5,2,1 represent dominant endomorphs). The subjects who do regular physical exercise or have cardiovascular, respiratory and musculoskeletal disorders, as well as active smokers, were excluded from the study.
Somatotype determination
The most popular approach for determining a person’s somatotype is the Heath-Carter somatotyping method. All participants were subjected to the following anthropometric measurements to determine their somatotype: Height, body weight, four skinfold thicknesses (triceps, subscapular, supraspinal and medial calf), two bone breadths (biepicondylar humerus and femur) and two limb girths (arm flexed and tensed calf).[2]
A small sliding calliper (Dasqua Monoblock 1380 Series [0–150 mm], UK) was used to measure bone breadths. A Harpenden calliper (British Indicators, c/o Assist Creative Resources, Wrexham, UK) was used to measure skinfold thicknesses, which can read to 0.1 mm by interpolation. The girths of the limbs were measured using fibreglass tape (Zyouu’s Tailor Inch [150 cm], In).
The somatotype components were derived using the equations given in the Heath-Carter somatotyping method.[2]
Measurement of cardiopulmonary parameters
Cardiopulmonary exercise test parameters were measured according to ATS guidelines using the Cosmed Quark breath-by-breath CPET system (Cosmed, Italy).[8] The goal of CPET was to implement an exercise program with progressively higher intensities until fatigue and the onset of limiting symptoms and indications. On a treadmill (Trackmaster, Full Vision Inc., USA), participants underwent a symptom-limited exercise test using the Bruce protocol. During the test, 12 lead electrocardiograms were recorded. The oxygen saturation of the blood was measured using a pulse oximeter placed on the finger. The participant wore a reusable oro-nasal mask during testing and breathed through a flow meter to measure breath-by-breath ventilation (VE) (L/min). A gas analyser and an open-circuit sampling system were used to calculate gas exchange. The test began with a 3-minute warm-up phase at 2.7 km/h and 0 % inclination, which was followed by the Bruce Protocol. It was terminated when the individual was unable to continue the exercise due to limiting symptoms such as fatigue, dyspnoea and pain in the legs. Following the exercise session, there was a 2-minute recovery period at the speed of 2 km/h with 0 % inclination. At the end of the exercise, a modified Borg dyspnoea scale was used to assess breathlessness.
The following parameters were measured using Omnia software: peak oxygen uptake (V̇O2), peak VO2/kg, metabolic equivalent (METS) in exercise testing, respiratory quotient (VCO2/VO2), VO2 at anaerobic threshold (VAT), minute ventilation (V.E), breathing reserve (BR), tidal volume (VT), O2 pulse, ventilatory equivalent for oxygen (VE/VO2), ventilatory equivalent for carbon dioxide (VE/ VCO2), heart rate (HR), HR reserve, HR recovery in 1min, end-tidal partial pressure of carbon dioxide, end-tidal partial pressure of oxygen, respiratory compensation and oxygen uptake efficiency slope (OUES).
Statistical analysis
The Shapiro–Wilk test was used to assess the distribution of the data. A comparison was done among somatotypes using the one-way analysis of variance test and the Kruskal–Wallis test for parametric and non-parametric data, respectively. Based on distribution, the data are provided as mean ± standard deviation or median (min. to max. range). The results were considered significant when P < 0.05. GraphPad Prism 9.0 (GraphPad Software, San Diego, California) software was used for the statistical analysis.
RESULTS
Demographic profile of subjects
Young males with a mean age of 24.10 ± 4.55 years (n = 50) took part in the study. Table 1 presents the demographic information as well as the parameters required to calculate an individual’s somatotype, duration of exercise and modified Borg dyspnoea scale. Age was comparable among the somatotypes. Ectomorphs were significantly taller than endomorphs and mesomorphs. In terms of weight, endomorphs were significantly heavier than ectomorphs. As compared to mesomorphs and ectomorphs, endomorphs had significantly higher triceps, subscapular, supraspinal and calf skinfold thickness. Mesomorphs had significantly thicker subscapular skinfolds than ectomorphs do and significantly greater humerus breadth than endomorphs and ectomorphs. Arm and calf girths were significantly lower in ectomorphs than in endomorphs and mesomorphs.
Parameters of somatotype | Endomorphs (n=20) | Mesomorphs (n=15) | Ectomorphs (n=15) | P-value |
---|---|---|---|---|
Age (yrs.) | 26.00 (18.00–34.00) | 25.00 (18.00–33.00) | 21.00 (18.00–34.00) | P=0.07 |
Height (cm) | 168.3 (161.3–184.9) | 167.5 (154–177) | 178.8 (165.8–190.5) | Pb=0.0104 Pc=0.0018 |
Weight (kg) | 74.03±11.51 | 69.74±8.90 | 61.31±8.92 | Pb=0.0004 |
Triceps skinfold (mm) | 19.84±4.338 | 13.92±4.536 | 10.59±3.231 | Pa=0.0001 Pb<0.0001 |
Subscapular skinfold (mm) | 19.24±4.135 | 15.04±4.170 | 9.906±3.144 | Pa=0.0044 Pb<0.0001 Pc=0.0010 |
Supraspinale skinfold (mm) | 16.15±4.077 | 9.814±2.183 | 7.156±2.277 | Pa<0.0001 Pb<0.0001 |
Calf skinfold (mm) | 16.20 (9.800–27.00) | 10.50 (6.200–18.60) | 11.50 (6.800–17.20) | Pa=0.0002 Pb=0.0006 |
Humerus breadth (cm) | 6.629±0.3986 | 6.935±0.4295 | 6.596±0.2605 | Pa=0.0372 Pc=0.0339 |
Femur breadth (cm) | 7.885±0.5176 | 8.113±0.9032 | 7.824±0.5727 | P=0.4156 |
Arm girth (cm) | 32.55 (28.20–39.70) | 33.10 (29.00–36.50) | 27.65 (21.80–29.50) | Pb<0.0001 Pc<0.0001 |
Calf girth (cm) | 36.84±2.852 | 36.91±2.430 | 33.53±2.055 | Pb=0.0002 Pc=0.0010 |
Modified Borg dyspnoea scale | 3.00 (0.50–6.00) | 2.00 (0.50–5.00) | 2.00 (0.50–4.00) | P=0.41 |
Mean duration of exercise (min: sec) | 07:46 | 08:54 | 09:35 |
CPET finding in different somatotypes
We examined the absolute values and percentage predicted values of CPET parameters in various somatotypes, as shown in Table 2 and Figures 1-3. We found that mesomorphs significantly outperformed endomorphs in terms of peak VO2, peak VO2 %predicted, peak VO2/kg, peak VO2/kg %predicted, METS, METS %predicted, V.E, oxygen pulse (O2 pulse) and VE/VO2 values. In contrast, ectomorphs significantly outperformed endomorphs in terms of peak VO2/kg and METS values. Mesomorphs achieved significantly lower maximum HR during exercise than ectomorphs and had significantly smaller BR than endomorphs and ectomorphs but significantly higher %predicted O2 pulse compared to endomorphs and ectomorphs. Other parameters were comparable.
CPET parameters | Endomorphs (n=20) | Mesomorphs (n=15) | Ectomorphs (n=15) | P-value |
---|---|---|---|---|
Peak oxygen consumption (mL/min) | 2151±287.8 | 2487±364.3 | 2342±403.8 | Pa=0.01 |
Peak oxygen consumption (%predicted) | 66.96±10.11 | 78.13±11.95 | 70.06±8.18 | Pa=0.003 |
Peak oxygen consumption/kg | 30.11±5.52 | 36.04±6.16 | 38.32±5.38 | Pa=0.0057 Pb<0.0001 |
Peak oxygen consumption/kg (%predicted) | 66.96±10.11 | 78.13±11.95 | 70.06±8.18 | Pa=0.003 |
METS | 8.77±1.86 | 10.29±1.74 | 10.95±1.54 | Pa=0.026 Pb=0.0004 |
METS %predicted | 66.92±10.09 | 78.13±11.95 | 70.06±8.18 | Pa=0.003 |
RQ | 1.27 (1.05–1.58) | 1.31 (1.15–1.58) | 1.26 (1.09–1.44) | P=0.80 |
BR | 53.80±17.16 | 38.38±11.87 | 52.79±9.47 | Pa=0.004 Pc=0.001 |
Tidal volume (L (btps)) | 1.78±0.50 | 2.03±0.41 | 1.99±0.40 | P=0.17 |
V.E | 71.13±25.39 | 95.32±18.63 | 80.29±14.43 | Pa=0.002 |
Respiratory frequency (1/min) | 38.19±6.89 | 48.25±11.34 | 40.92±7.03 | Pa=0.001 Pc=0.03 |
O2 pulse (mL/beat) | 12.80 (9.20–21.90) | 14.80 (10.30–24.00) | 12.85 (10.10–18.50) | Pa=0.02 |
O2 pulse %predicted | 77.50 (51.00–115.00) | 90.00 (67.00–147.00) | 76.50 (17.60–98.00) | Pa=0.006 Pc=0.033 |
HR (bpm) | 171.5 (142.00–194.00) | 166.00 (125.00–186.00) | 178.00 (159.00–189.00) | Pc=0.04 |
HR % predicted | 87.23±8.673 | 82.67±10.55 | 88.94±5.418 | P=0.097 |
HRR (bpm) | 23.00 (5.00–54.00) | 20.50 (9.00–55.00) | 17.00 (4.00–36.00) | P>0.99 |
VE/VO2 | 34.24±5.99 | 39.40±7.02 | 35.80±5.82 | Pa=0.03 |
VE/VCO2 | 27.45 (19.50–42.40) | 29.10 (25.60–40.50) | 26.55 (23.00–34.50) | P=0.17 |
OUES | 2550±422.9 | 2647±414.2 | 2645±447.8 | P=0.70 |
OUES (%predicted) | 81.00±14.04 | 83.80±10.01 | 83.72±12.23 | P=0.70 |
VO2 at anaerobic threshold (mL/min) | 1686±406.1 | 1651±326.2 | 1795±382.5 | P=0.51 |
VO2 at anaerobic threshold (%pred) | 75.40±16.02 | 66.73±11.02 | 76.89±13.93 | P=0.09 |
End-tidal PO2 (PetO2) | 105.7±6.41 | 109.7±4.60 | 106.2±4.60 | P=0.07 |
End-tidal PCO2 (PetCO2) | 44.04±6.26 | 39.87±4.82 | 42.78±4.50 | P=0.06 |
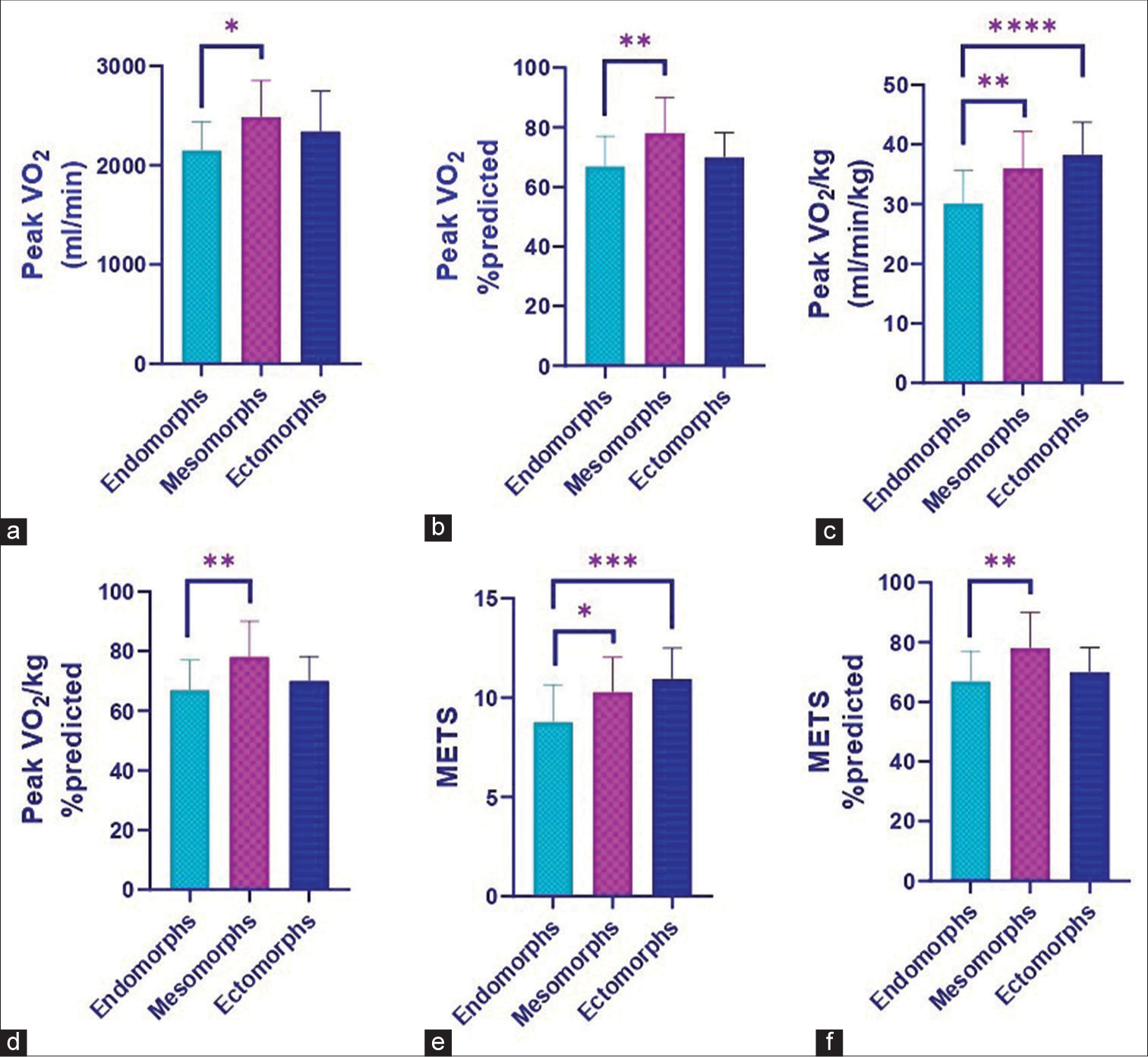
- The graphs depicting cardiopulmonary exercise parameters in different somatotypes: (a) Peak VO2 (b) Peak VO2 %predicted (c) Peak VO2/kg (d) Peak VO2/kg % predicted (e) METS (f) METS % predicted Values are plotted as mean ± standard deviation or median (minimum to maximum). *P < 0.05, **P < 0.01 and ***P < 0.001, ****P ≤ 0.0001 for inter group comparison. VO2: Peak oxygen consumption; METS: Metabolic equivalent in exercise testing.
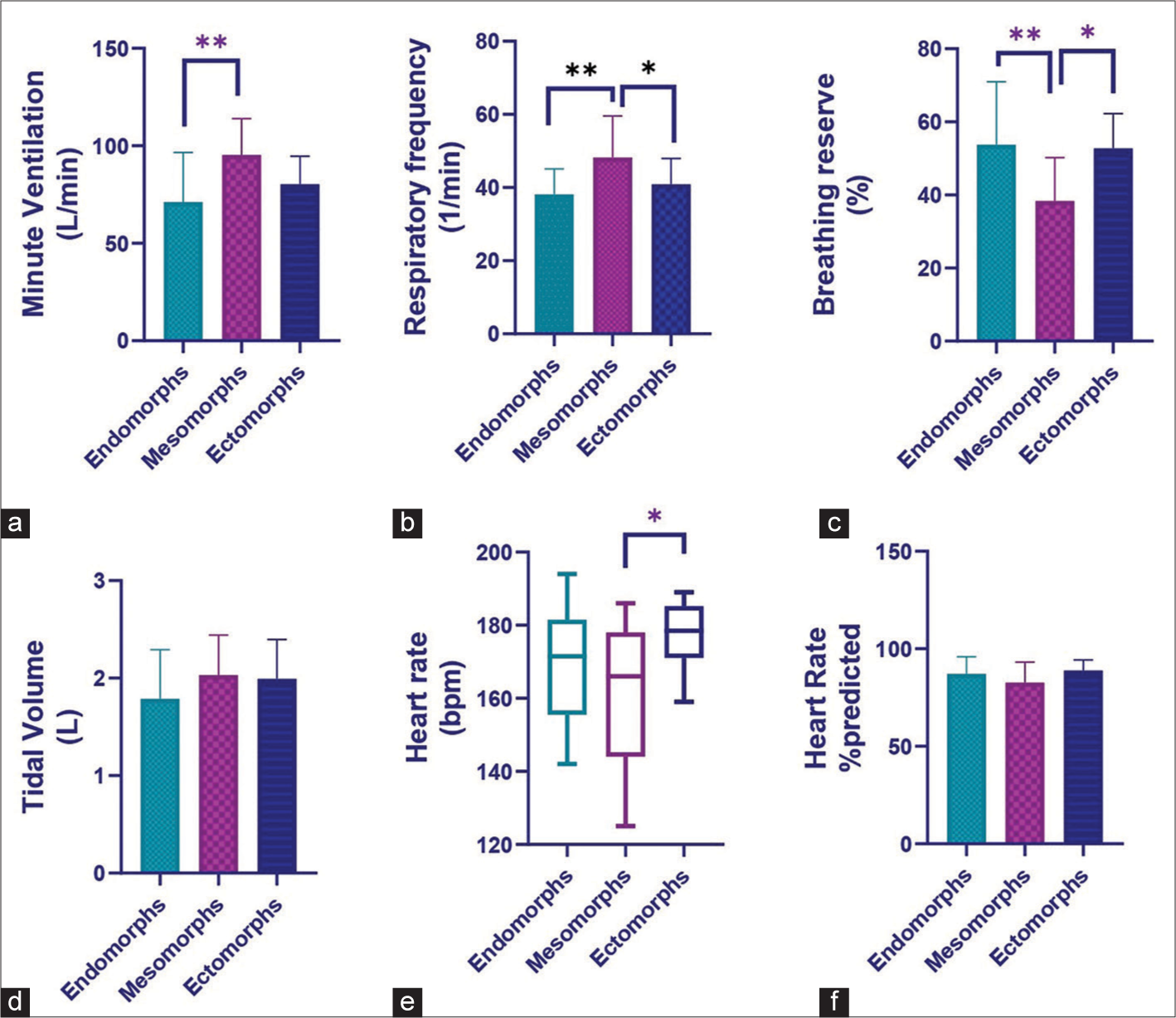
- The graphs depicting the cardiopulmonary exercise parameters in different somatotypes: (a) Minute ventilation, (b) Respiratory frequency (c) Breathing reserve (d) Tidal volume, (e) Heart rate, (f) Heart rate % predicted. Values are plotted as mean ± standard deviation or median (minimum to maximum). *P < 0.05, **P < 0.01 for inter group comparison.
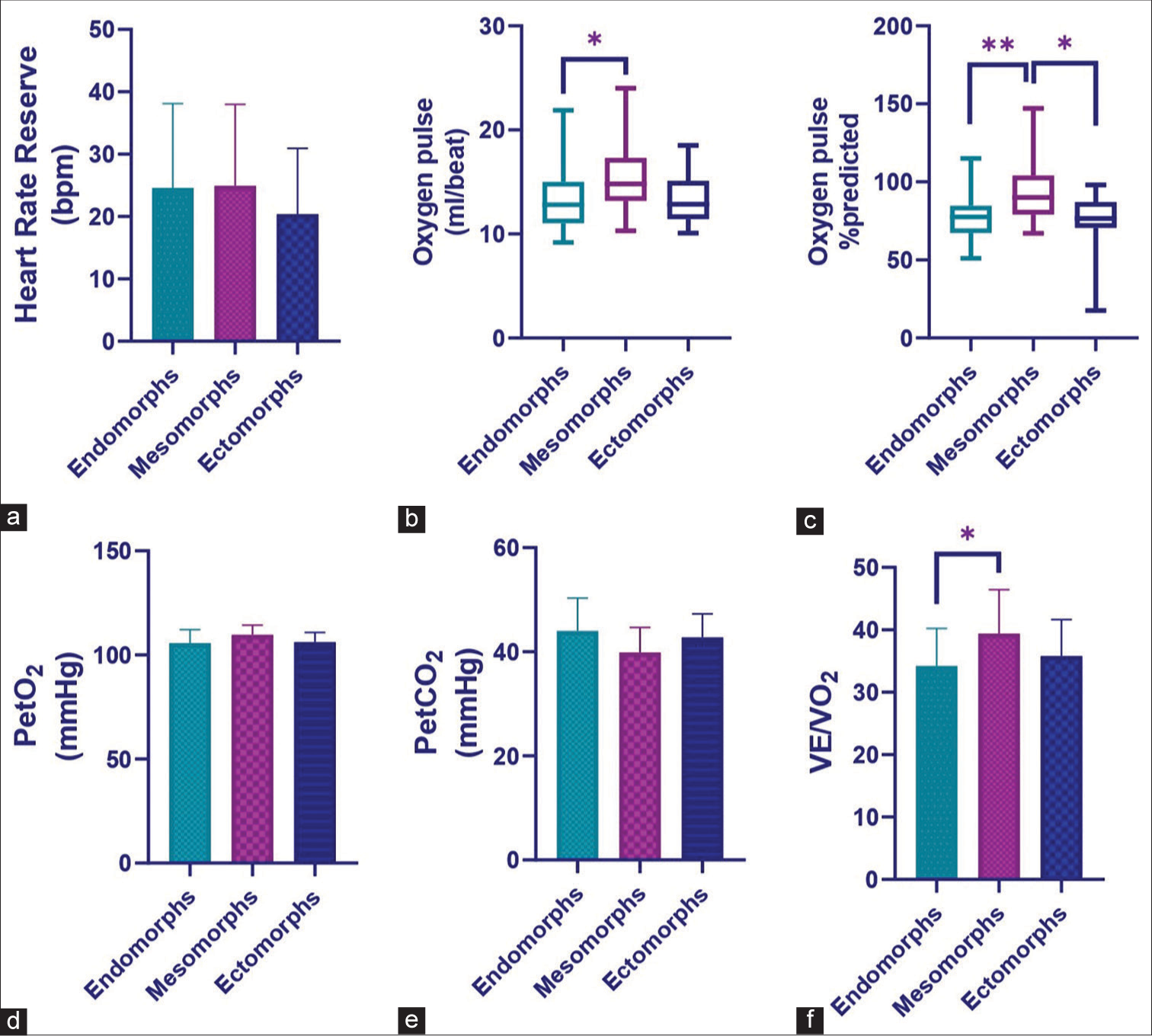
- The graphs depicting the cardiopulmonary exercise parameters in different somatotypes: (a) Heart rate reserve (b) Oxygen pulse (c) Oxygen pulse % predicted (d) PetO2 (e) PetCO2 (f) VE/ VO2. Values are plotted as mean ± standard deviation or median (minimum to maximum). *P < 0.05, **P < 0.01 and for inter group comparison. petO2: End-tidal PO2; PetCO2: End-tidal PCO2; VE/VO2: Ventilatory equivalent for oxygen.
Correlation between determinants of somatotype and the parameters of CPET
We investigated the correlation between somatotype determinants and the cardiopulmonary exercise test parameters listed in Table 3 and Figure 4. Weight had a positive correlation with O2 pulse and OUES. BR, VO2 at anaerobic threshold, VO2 at anaerobic threshold %predicted, and OUES all showed positive correlations with height. Humerus breadth was positively correlated with OUES. Arm girth was inversely associated with HR but positively correlated with O2 pulse and VE/VCO2 slope. Calf girth was positively correlated with oxygen pulse but negatively correlated with peak VO2/kg, METS, breathing reserve, heart rate and heart rate % predicted.
Spearman correlation | Weight | Height | Humerus breadth | Arm girth | Calf girth |
---|---|---|---|---|---|
Peak VO2/kg | r=−0.55 | r=0.02 | r=−0.11 | r=−0.49 | r=−0.37 |
P=5.48 | P=0.86 | P=0.40 | P=7.73 | P=0.003 | |
METS | r=−0.50 | r=0.06 | r=−0.05 | r=−0.48 | r=−0.33 |
P=3.82 | P=0.62 | P=0.70 | P=1.08 | P=0.009 | |
BR | r=0.03 | r=0.39 | r=0.01 | r=−0.22 | r=−0.10 |
P=0.77 | P=0.002 | P=0.91 | P=0.08 | P=0.45 | |
HR | r=−0.18 | r=0.12 | r=−0.17 | r=−0.35 | r=−0.34 |
P=0.16 | P=0.28 | P=0.18 | P=0.005 | P=0.007 | |
HR %pred | r=−0.03 | r=−0.11 | r=−0.16 | r=−0.19 | r=−0.26 |
P=0.79 | P=0.33 | P=0.22 | P=0.14 | P=0.04 | |
VO2/HR | r=0.29 | r=0.14 | r=0.21 | r=0.26 | r=0.29 |
P=0.02 | P=0.26 | P=0.09 | P=0.04 | P=0.02 | |
VO2 at AT | r=0.24 | r=0.36 | r=0.13 | r=0.05 | r=0.15 |
P=0.06 | P=0.004 | P=0.29 | P=0.69 | P=0.23 | |
VO2 at AT %pred | r=0.15 | r=0.27 | r=0.07 | r=3.80 | r=0.09 |
P=0.24 | P=0.03 | P=0.55 | P=0.99 | P=0.47 | |
Respiratory compensation | r=0.29 | r=0.17 | r=0.01 | r=0.22 | r=0.15 |
P=0.02 | P=0.18 | P=0.89 | P=0.08 | P=0.23 | |
VE/VCO2 slope | r=0.11 | r=−0.14 | r=0.08 | r=0.29 | r=0.17 |
P=0.40 | P=0.27 | P=0.54 | P=0.02 | P=0.18 | |
OUES | r=0.32 | r=0.35 | r=0.27 | r=0.12 | r=0.27 |
P=0.01 | P=0.006 | P=0.03 | P=0.33 | P=0.03 |
In this correlation, P values and r-values are presented, and the bold text represents a significant correlation analysed by the Spearman correlation test. METS: Metabolic equivalent, HR: Heart rate, BR: Breathing reserve, AT: Anaerobic threshold, VE/VCO2: Ventilatory equivalent for carbon dioxide, OUES: Oxygen uptake efficiency slope, VO2: Oxygen consumption
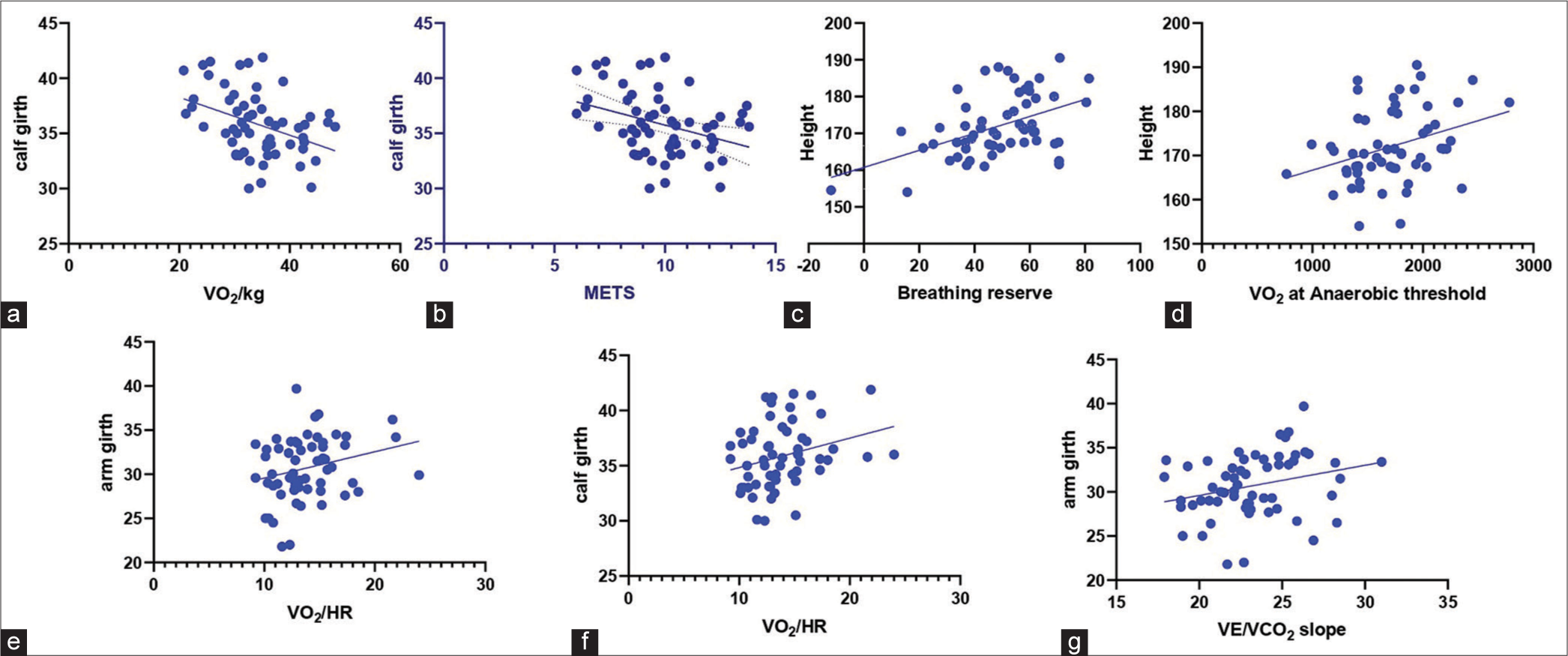
- The graphs show correlation between somatotype dimensions and cardiopulmonary exercise test parameters. Calf girth correlates negatively with (a) peak VO2/kg and (b) METS. Height correlates positively with (c) breathing reserve and (d) VO2 at anaerobic threshold. Arm girth correlates positively with (e) VO2/HR. Calf girth correlates positively with (f) VO2/HR. Arm girth correlates positively with (g) VE/VCO2 slope.
DISCUSSION
In the present study, cardiorespiratory responses to incremental exercise have been investigated in young adults having dominant somatotypes.
Compared to other approaches, the Heath and Carter method is a better predictor of body composition since it accounts for variables including height, weight, skinfold thickness, bone width and limb girths. This approach offers more precise information on body composition than BMI and waist-hip ratio alone.
Mesomorphs having higher muscle mass compared to endomorphs may explain their significantly higher peak VO2, peak VO2% predicted, peak VO2/kg and peak VO2/kg% predicted compared to endomorphs. Imms et al. have reported greater oxygen consumption during contraction of the large mass muscle (quadriceps) than that during small mass muscle (hand gripping) at 20% maximum voluntary contraction.[9] The significantly lower values in endomorphs may be explained by their comparatively slower metabolism, which causes more fat accumulation in the body. Pourhassan et al. studied the relationship between submaximal oxygen uptake and body composition and reported a strong association between VO2submax and fat-free mass (FFM) and concluded that FFM is the determinant of VO2 submax.[10] Saha has determined the impact of somatic and body composition variables on aerobic capacity in different somatotypes. She observed a negative correlation between VO2 max and body fat percentage, body surface area and endomorphy component of somatotype, while it correlated positively with the lean body mass, percentage of skeletal muscle mass and mesomorphy component of somatotype.[11] Oda et al. also reported a significant negative correlation between peak oxygen uptake with total body fat percentage.[12] These findings suggest that VO2max is influenced by the total active muscle mass being recruited during exercise, where the more muscle mass is involved during exercise, the higher the VO2max.[13]
A MET is the quantity of oxygen consumed while at rest, the value of which is around 3.5 mL O2/kg/min (1.2 kcal/min for a person weighing 70 kg). The MET concept offers a straightforward, understandable method for describing the energy expenditure of physical activity as a multiple of resting metabolic rate. The energy cost of an activity may be calculated by dividing the relative oxygen cost of the activity (ml O2/kg/min) by 3.5.[14] In the present study, METS values were significantly lower in endomorphs than other somatotypes for maximum physical activity. The amount of skeletal muscle is a key factor in the rise in metabolism during exercise and the absolute maximum oxygen consumption. Lean tissue has a significant relationship with basal metabolism, and skeletal muscles are the source of energy used during physical activity.[15] This might be the reason why mesomorphs achieved higher METS than endomorphs. Thus, higher VO2peak/kg and METS values observed in mesomorphs may be explained by the higher muscle mass in these individuals.
In an exercising person, maximal oxygen uptake is limited by the ability of the cardiorespiratory system to deliver oxygen to the exercising muscles.[16] The increase in the O2 pulse after the training session may reflect the degree of peripheral and cardiac adaptation to training. In our study, we observed that O2 pulse (the amount of oxygen consumed per heartbeat during exercise), which can be used as an indirect indicator of cardiac stroke volume,[17] was significantly higher in mesomorphs as compared to endomorphs. Chaouachi et al. have also reported higher values of O2 pulse in mesomorphs in comparison to the endomorphs and ectomorphs’ groups.[18] We also observed that O2 pulse significantly correlated with arm and calf girths. The calf muscle pump plays a very important role in increasing venous return, which can also affect the cardiac output.
We found that mesomorphs had significantly higher V.E than endomorphs, and respiratory frequency was significantly higher compared to endomorphs and ectomorphs, but the BR was significantly lower as compared to endomorphs and ectomorphs. While exercising, VTs and respiratory rates are increased, increasing oxygen consumption. This is generally achieved by raising the respiratory rate because VTs are frequently not raised. Uneven body fat distribution may restrict diaphragmatic excursion and impede further VT augmentation during exercise.[19] Mesomorphs have a significantly smaller BR, which may be related to their increased V.E.
Subhan et al. reported that there is no significant difference in maximal HR achieved during exercise among persons who are overweight, normal weight and obese as determined by BMI.[20] Nevertheless, we noticed that ectomorphs achieved significantly higher maximal HR during exercise than mesomorphs. The reason behind this is not yet clear.
High airway resistance might be the cause of the VE/VO2 and VE/VCO2 not rising with maximum activity.[21] We found that VE/VO2 was significantly higher in mesomorphs than in endomorphs. This may be a result of their significantly higher respiratory frequency and V.E. One of the possibilities might be that endomorphs have a significantly higher peripheral airway resistance, leading to a decreased VE/VO2 ratio.
Mesomorphs have a higher percentage of muscular components, and endomorphs have relatively more body fat than mesomorphs.[22] Excess body fat may exert an unfavourable burden and a hindering action on cardiac function, particularly during exhausting exercise when excessively hyperactive body muscles fail to uptake enough oxygen due to the deposition of a proportionately higher amount of fat mass. Extra body fat affects mechanical efficiency for a given workload and impairs cardiorespiratory functions.[23]
CONCLUSION
Mesomorphs have higher peak oxygen consumption than endomorphs, along with higher METS, V.E and O2 pulse. Clinicians should consider somatotype when interpreting the results since, even though all the participants were in good health, there is still variation in their cardiorespiratory responses.
Ethical approval
This study was approved by the Institute Ethics Committee for Human Subjects (Ref. No.: IECPG-520/23.09.2021, RT-02/28.10.2021 dated 29.10.21).
Declaration of patient consent
The authors certify that they have obtained all appropriate patient consent.
Conflicts of interest
There are no conflicts of interest.
Use of artificial intelligence (AI)-assisted technology for manuscript preparation
The authors confirm that there was no use of artificial intelligence (AI)-assisted technology for assisting in the writing or editing of the manuscript and no images were manipulated using AI.
Financial support and sponsorship
Nil.
References
- Somatotyping by physical anthropometry. Am J Phys Anthropol. 1954;12:209-39.
- [CrossRef] [PubMed] [Google Scholar]
- The heath-carter anthropometric somatotype - instruction manual-2002. Available from: https://www.semanticscholar.org/paper/the-heath-carter-anthropometric-somatotypemanual-carter/13bf62be916b98b2b05b3297f7bf4ca11f6c09b3 [Last accessed on 2023 Nov 02]
- [Google Scholar]
- Somatotyping: Development and applications Cambridge, UK: Cambridge University Press; 1990. p. :524.
- [Google Scholar]
- Chapter 23: Anthropometric twin studies In: Tarnoki A, Tarnoki D, Harris J, Segal N, eds. Twin research for everyone. United States: Academic Press; 2022. p. :387-402. Available from: https://www.sciencedirect.com/science/article/pii/B9780128215142000246 [Last accessed on 2024 Aug 31]
- [CrossRef] [Google Scholar]
- Association of dominant somatotype of men with body structure, function during exercise, and nutritional assessment. Am J Hum Biol. 2000;12:167-80.
- [CrossRef] [Google Scholar]
- Anaerobic capacity as a function of somatotype and participation in varsity athletics. Res Q. 1973;44:197-205.
- [CrossRef] [PubMed] [Google Scholar]
- Cardiopulmonary exercise testing and its application. Postgrad Med J. 2007;83:675-82.
- [CrossRef] [Google Scholar]
- ATS/ACCP Statement on cardiopulmonary exercise testing. Am J Respir Crit Care Med. 2003;167:211-77.
- [CrossRef] [PubMed] [Google Scholar]
- Respiratory responses to sustained isometric muscle contractions in man: The effect of muscle mass. J Physiol. 1989;419:1-14.
- [CrossRef] [PubMed] [Google Scholar]
- Relationship between submaximal oxygen uptake, detailed body composition, and resting energy expenditure in overweight subjects. Am J Hum Biol. 2015;27:397-406.
- [CrossRef] [PubMed] [Google Scholar]
- Somatic and body composition factors underlying aerobic capacity. Am J Sports Sci. 2015;3:36.
- [CrossRef] [Google Scholar]
- Relationship between peak oxygen uptake and regional body composition in Japanese subjects. J Sport Health Sci. 2014;3:233-8.
- [CrossRef] [Google Scholar]
- The effect of aging on relationships between lean body mass and VO2max in rowers. PLoS One. 2016;11:e0160275.
- [CrossRef] [PubMed] [Google Scholar]
- Metabolic equivalents (METS) in exercise testing, exercise prescription, and evaluation of functional capacity. Clin Cardiol. 1990;13:555-65.
- [CrossRef] [PubMed] [Google Scholar]
- Metabolic equivalents of task are confounded by adiposity, which disturbs objective measurement of physical activity. Front Physiol. 2015;6:226.
- [CrossRef] [PubMed] [Google Scholar]
- Limiting factors for maximum oxygen uptake and determinants of endurance performance. Med Sci Sports Exerc. 2000;32:70-84.
- [CrossRef] [PubMed] [Google Scholar]
- Oxygen pulse. 2014 Available from: https://academic.oup.com/book/24372/chapter/187250763 [Last accessed on 2022 Jul 27]
- [CrossRef] [Google Scholar]
- Effects of dominant somatotype on aerobic capacity trainability. Br J Sports Med. 2005;39:954-9.
- [CrossRef] [PubMed] [Google Scholar]
- Impact of obesity on respiratory function. Respirology. 2012;17:43-9.
- [CrossRef] [PubMed] [Google Scholar]
- Underweight and overweight men have greater exercise-induced dyspnoea than normal weight men. Ups J Med Sci. 2012;117:383-9.
- [CrossRef] [PubMed] [Google Scholar]
- Cardiopulmonary exercise testing in the assessment of exertional dyspnea. Ann Thorac Med. 2015;10:77-86.
- [CrossRef] [PubMed] [Google Scholar]
- Correlation of maximum oxygen consumption with component composition of the body, body mass of men with different somatotypes aged 25-35. Pedagogy Phys Cult Sports. 2020;24:290-6.
- [CrossRef] [Google Scholar]
- Maximal oxygen intake and its relation to body composition, with special reference to chronic physical activity and obesity. J Appl Physiol. 1957;11:72-8.
- [CrossRef] [PubMed] [Google Scholar]