Translate this page into:
Biomechanical responses on specific load carriage and positive gradient walking: A pilot study
*Corresponding author: Madhusudan Pal, PhD Director and Scientist G, Centre of Excellence (CoEs), Footwear Design and Development Institute (FDDI), A-10/A, Sector 24, Noida 201301, India. Ministry of Commerce and Industry, Government of India. madhusudanpal@rediffmail.com
-
Received: ,
Accepted: ,
How to cite this article: Kaharwar VS, Kumar SD, Pal M. Biomechanical responses on specific load carriage and positive gradient walking: A pilot study. Indian J Physiol Pharmacol. 2024;68:25-32. doi: 10.25259/IJPP_393_2023
Abstract
Objectives:
Normal load carriage is an inevitable part of military marching tasks. Military tasks are inextricably related to carrying huge loads irrespective of different terrain. Continuous carrying of heavy loads from level ground to uphill gradient may alter kinetic and kinematic responses. Such responses, in the long run, may cause the risk of injury. This study was designed to find out the effects of external load on kinetic and kinematic responses at specific loads and grades (+10°).
Materials and Methods:
Six healthy Indian soldiers mean (± standard error of mean [SEM]) age 30.5 ± 3.5 years, height 168.7 ± 2.8 cm, weight 73.8 ± 7.08 kg participated in this study, walking on treadmill (Deneb and Polak-speed-1625) at 3.5 kmph for 6 min at 10° inclination for two conditions, with no-load and 30 kg compact-load at controlled laboratory condition 25°C and 50% relative humidity (RH). Compressive, shearing, torque, joint reaction force, and erector-spine forces for kinetic and angular changes of neck, trunk, thigh, forearm, upper arm, and leg were analysed by biomechanical analysis software (Ergomaster 4.6). A paired t-test and repeated measures analysis of variance were applied to determine the significant effects of the load on dependent variables.
Results:
Significant changes were found in kinetic (compressive, shearing, torque, joint reactive force, and erector spine force) and kinematic (neck and trunk) parameters with subsequent increments of loads.
Conclusion:
From this study, it was concluded that walking in uphill condition, compressive, shearing, torque, joint reactive force, and erector spine force was found to be increased by 2.58, 4.65, 4.06, 2.83, and 4.06 folds, the angular changes found in neck and trunk were 1.78 and 1.25 folds compared to no-load conditions, respectively. The exerted forces, namely compressive force 74.12%, shearing force 86%, joint reaction force almost 75%, and erector spine force 83.82%, were very close to the injury risk profile; only the change of torque was not much closer to the risk profile. Such findings could be used for recommending load carriage guidelines for future studies.
Keywords
Uphill gradient
Kinetic
Kinematic responses
Load carriage
Injury risk
INTRODUCTION
The military profession is inextricably linked to the activity of marching tasks. During load marching tasks, military personnel generally carry heavy loads while marching long distances. Carrying heavy loads for a longer duration might cause multiple injuries.
Military personnel cannot swing their arms normally as they walk carrying a rifle and a backpack that together weigh roughly around 40 kg. The weight of the ensembles together causes the impact peak force to increase, which constraint upper body movements.[1]
Few literatures suggest that the accumulation of impact pressure is unquestionably a factor in the progress of overuse damage of the lower limb. The addition of outside loads will need the bearer to respond by changing their posture and gait mechanics.[2] A person’s gait mechanics and postural stability change when an external load is added to them because it shifts the body’s centre of mass (CoM). Forward leaning is a normal reaction to posterior weight-bearing with CoM changing.[3]
The body must adjust to changing CoM when a load is given posteriorly to the trunk, which is normally done by increasing trunk flexion. The size and placement of the loads are two elements that affect the magnitude of forward lean. Greater forward lean will be produced by a heavier load.[4]
The physiological effects of different load transport activities have been the subject of substantial investigation globally. But, only few literatures have been reported on the kinematics of gait alone, which focus primarily on military load carrying during level walking that are notable.[5-9] Considering kinetic and kinematic changes during military load carriages, there have only been a few studies conducted so far. Pal et al., recommended the ideal carrying load for soldiers while walking at specific speeds on level ground.[10]
According to a frequently cited study, any type of load should be carried at or below 30% of the bearer’s body weight unless it increases the risk of injury.[11] According to Chatterjee et al.,[12] up-hill walking at three kmph carrying a 24.1 kg load cannot be permitted beyond 10°. For continuous carriage of load during eight hours at a 15° incline, only 10.7 kg is allowed. 21.4 kg is allowed for only two hours at the same speed. However, carrying a load is either a requirement for employment in industry or a crucial component of military activities, as it may be challenging to maintain this threshold limit. It is crucial to comprehend the biomechanical and physiological changes of the human body while carrying huge loads. This will ensure that load carriage can be carried out securely and with the least amount of risk to the bearer.[4]
Extensive research data is available on physiological responses during load carriage at different gradient walking; however, data on biomechanical responses over different gradient walking are very limited. Under this circumstance, the present study was therefore designed to estimate the effect of external load on kinetic and kinematic responses at 10° uphill walking at two conditions: no-load and 30 kg compact load.
MATERIALS AND METHODS
Subjects
In total, six healthy male infantry soldiers were selected to volunteer for the study. Excluding criteria for subjects are as follows: any previous history of musculoskeletal disorders or fractures on body parts. Their mean (SEM) age, height, and weight were 30.5 ± 3.5 years, 168.7 ± 2.8 cm and 73.8 ± 7.08 kg, respectively. A minimum of five trials of each subject were taken for the experimental procedure (6 × 5 = 30 trials for six subjects) of each condition: 10° inclination with no load and with the load. Hence, for both conditions, the total trials of six subjects were 30 × 2 = 60 trials. During the data processing, seven trials were excluded from each condition due to diversity, and finally, (23+23 trials) were considered for each condition.
The necessary information regarding the experimental protocol was given to subjects before the commencement of the study, and they signed the informed consent.
The Institutional Ethical Committee approved the study protocol on human use as an experimental subject and the entire principles of the experiment outlined by the Declaration of Helsinki Protocol, 1964. This study was also approved by the Institutional Ethical Committee (Ref no: IEC/DIPAS/D-1/2, 21-22, dated 12.01.21) on the use of human as study subjects.
Experimental design
Initially, the participants were briefed about the objectives and protocol of the study. They needed to be habituated to walking on a treadmill (Deneb and Polok-Speed-1625) at an inclination in the controlled laboratory conditions with and without load at 3.5kmph walking speed. All participants were made to go through this habituation process.
After the habituation process, the participants were asked to perform treadmill walking for two conditions: no-load and 30 kg compact load at a constant walking speed of 3.5 kmph for the final experiment. 30 kg load was compacted in an existing military load carriage backpack.
The experiment was carried out inside the controlled laboratory with environmental factors maintained, where temperature was 25°C ± 3°C and relative humidity was 50 ± 3%. The uphill walking was performed at an incremental gradient of the treadmill at 10°. The duration of one trial per subject was 10 min. The total duration of the experiment was (5 trials × 1subject × 10 min = 50 min) 50 min × 6 subjects = 300 min at one condition. Initial 2 min and last 2 min data were excluded, and mid 6 min data were considered to maintain uniformity. 3–4 min resting time was offered to participants during five trials.
During the time of the study being performed, a DSLR camera was placed to capture the best postural videos and images throughout the experiment. Videographic imaging of body movements was done, and snapshots were taken at regular intervals. The selected photo ranges were 300 ± 20 px × 400 ± 20 px for final biomechanical assessment.
Parameters studied
Compressive force, shearing force, torque, joint reaction force, and erector spine force for kinetic analysis and kinematic analysis. The angular changes of the neck, thigh, forearm, upper arm, trunk, and leg were measured by biomechanical analysis software using ‘Ergomaster’ 4.6. An average of mid-6 min data was considered for statistical analysis.
Statistical analysis
Descriptive statistics of all selected trials were calculated. Data were represented as mean ± SEM. A paired t-test was calculated to find the significant changes between load and no-load conditions of different body joints. To find out the significant effect of load on gradient walking and to validate the paired t-test score, repeated measures analysis of variance (ANOVA) was done using the statistical software Minitab 20.4. All differences were considered significant at P < 0.05.
RESULTS
Kinetic responses, for example, compressive force, shearing force, torque, joint reaction forces, and erector spine forces, and kinematic responses, namely neck, trunk, thigh, upper arm, forearm, and leg during 30 kg load carriage and no-load condition with 10° incline walking are graphically presented in [Figures 1-3 and Table 1]. Repetitive measure ANOVA showed a significant load × gradients interaction on compressive force (F = 548.51, P < 0.05), shearing force (F = 698.94, P < 0.05), torque (F = 369.78, P < 0.05), joint reaction force (F = 630.88, P < 0.05), erector spine force (F = 369.78, P < 0.05), angular changes in neck (F = 71.43, P < 0.05) and trunk (F = 252.28, P < 0.05). No significant changes were found in the thigh, leg, upper-arm and forearm. The individual value plots [Figure 3a and b] to evaluate the spreads of the data to identify the outliers to determine whether the potential difference was statistically significant from the hypothesised mean of difference. The calculated value from the paired t-test of this study was <0.05, signifying rejection of the null hypothesis.
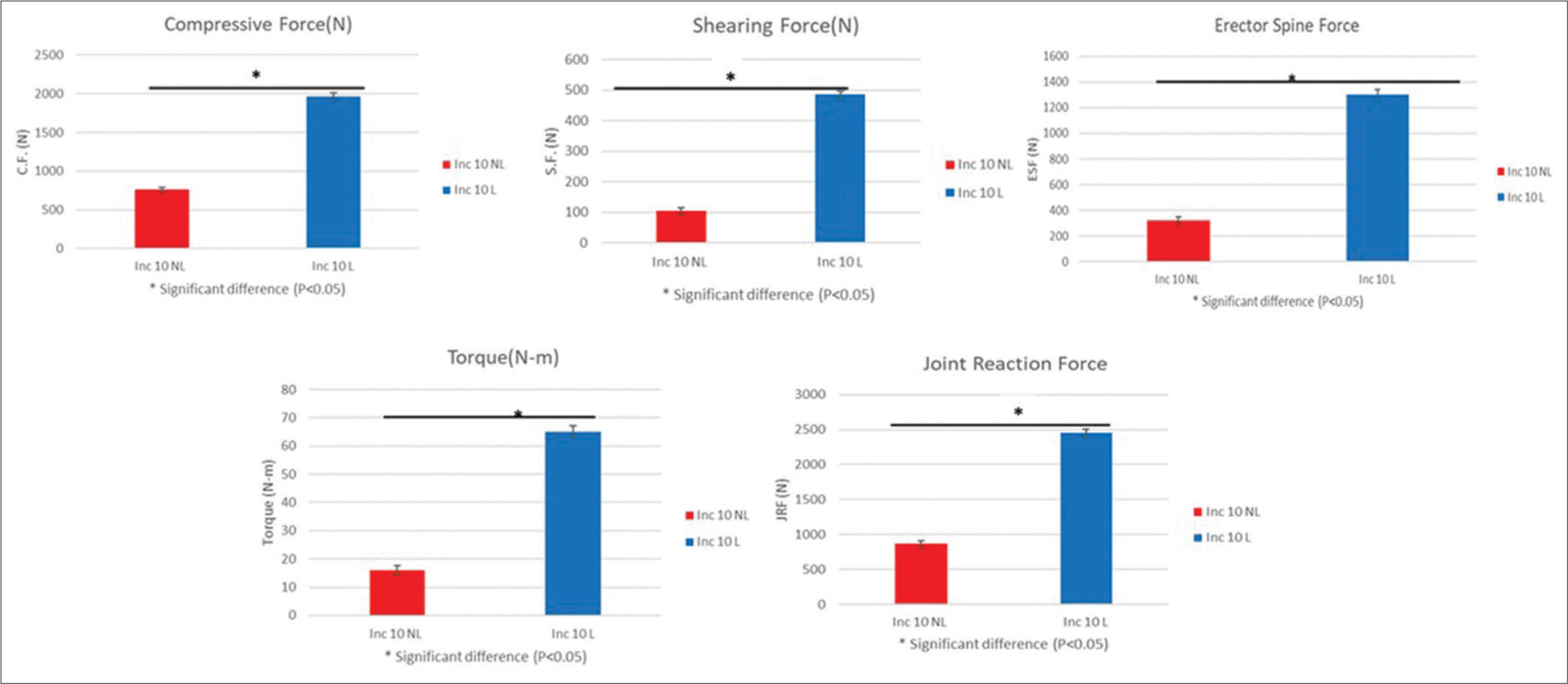
- Graphical representation of kinetic responses during treadmill walking at 3.5 kmph with carrying a load (30 kg) at a 10° gradient. Inc.: Inclination, NL: No-load, L: Load. Inc: Inclination, NL: No-load, L: Load, CF: Compressive force, SF: Shearing force, JRF: Joint reaction force, ESF: Erector spinae force. *Significant difference at 0.05 level.
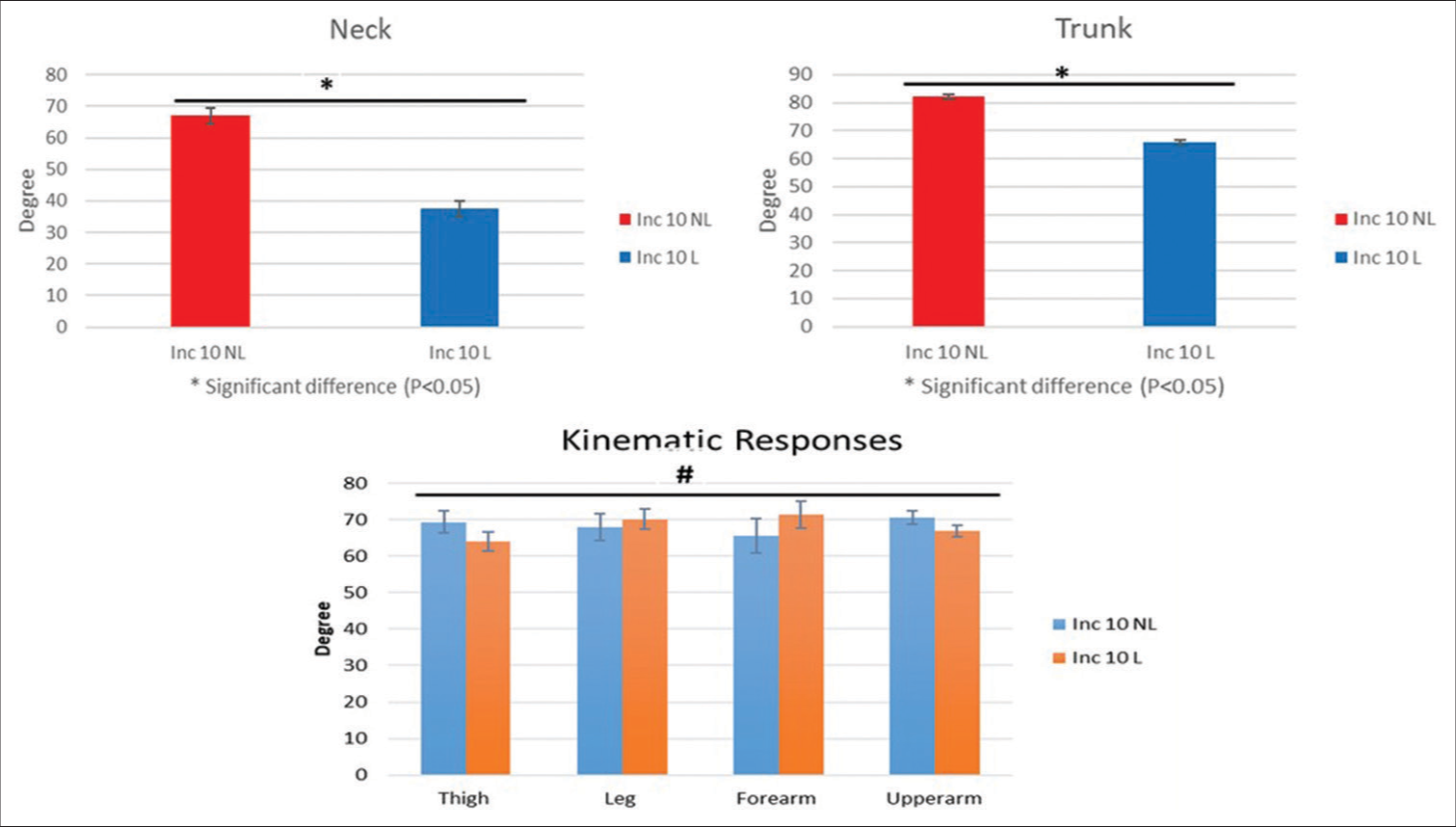
- Graphical representation of kinematic responses during treadmill walking at 3.5 kmph with carrying load (30 kg) at 10° gradient. Inc.: Inclination, NL: No-load, L; Load. *Significant difference at 0.05 level. #No significant difference.
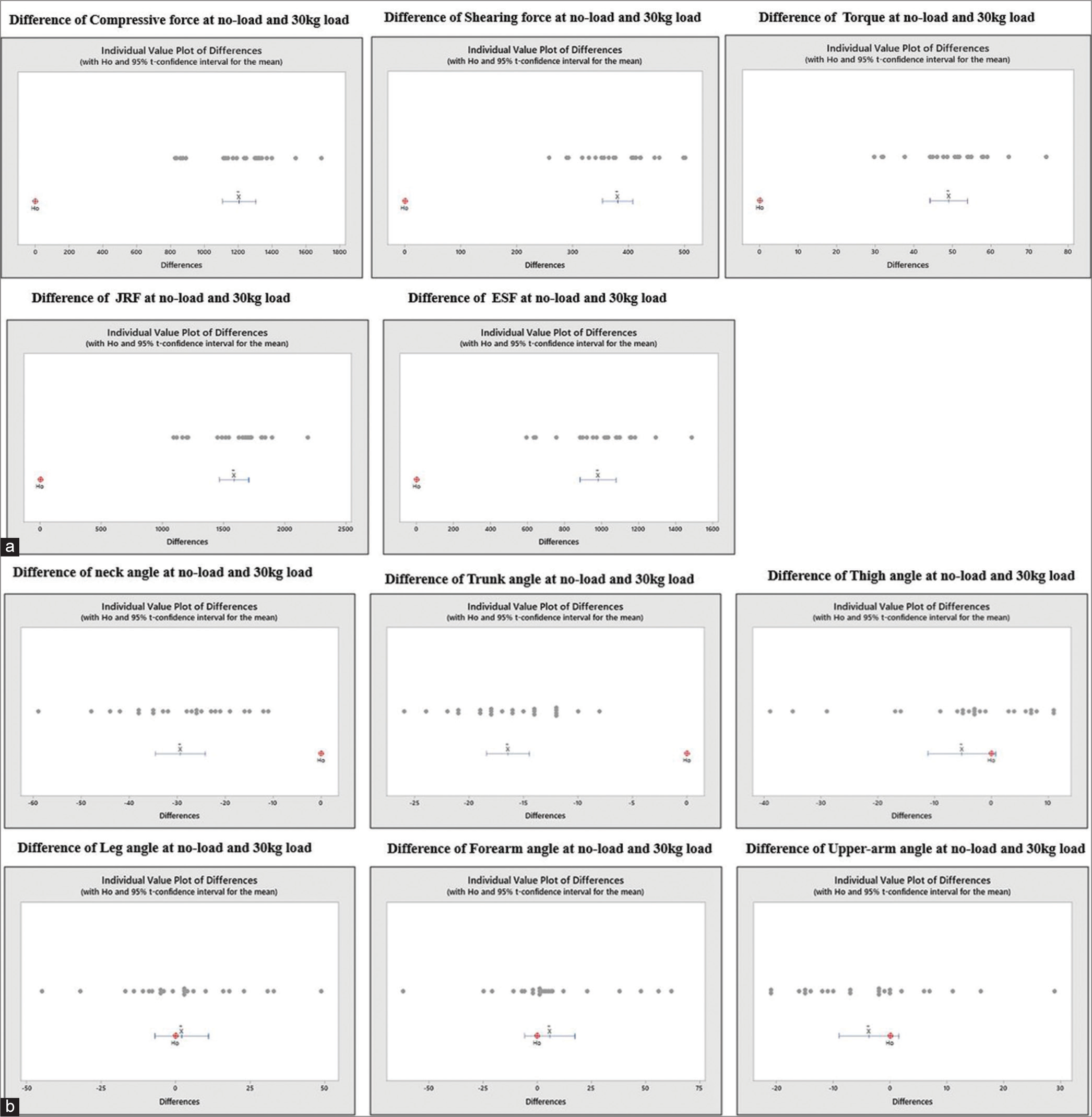
- (a) Graphical representation of individual value plots of kinetic parameters during treadmill walking at 3.5 kmph with carrying a load (30 kg) at 10° gradient and (b) graphical representation of Individual value plots of kinematic parameters during treadmill walking at 3.5 kmph with carrying a load (30 kg) at 10° gradient. JRF: Joint reaction force, ESF: Erector spinae force. Indication denotes: x-bar: 95%CI ranges, HO: Null hypothesis.
Parameter | Factors | Mean±SEM | Coef | P-value | |
---|---|---|---|---|---|
Inc 10°NL (95% CI) | Inc 10°NL (95% CI) | ||||
Kinetic | CF (N) | 762.3±28.13 (688.9, 835.6) |
1967.7±43.10 (1894.4, 2041.1) |
763.9 | 0.001 |
SF (N) | 104.2±9.81 (83.68, 124.72) |
484.9±10.53 (464.3, 505.4) |
104.70 | 0.008 | |
Torque (Nm) | 16.03±1.50 (12.40, 19.67) |
65.12±2.06 (61.48, 68.76) |
16.10 | 0.009 | |
JRF (N) | 866.5±37.69 (776.5, 956.5) |
2452.6±50.65 (2362.6, 2542.6) |
868.6 | 0.002 | |
ESF (N) | 320.7±30.00 (248.0, 393.5) |
1302.3±41.29 (1229.6, 1375.1) |
322.0 | 0.009 | |
Kinematic | Neck (°) | 67±2.54 (62.05, 71.95) |
37.65±2.36 (32.70, 42.60) |
68.71 | 0.000 |
Trunk (°) | 82.261±0.788 (80.786, 83.735) |
65.826±0.670 (64.352, 67.301) |
81.938 | 0.000 | |
Thigh (°) | 68.04±3.68 (61.44, 74.65) |
70.13±2.81 (63.52, 76.74) |
69.93 | 0.562 | |
Leg (°) | 69.30±3.04 (63.55, 75.06) |
64.08±2.65 (58.34, 69.84) |
67.75 | 0.203 | |
FA (°) | 65.56±4.72 (56.98, 74.15) |
71.34±3.73 (62.76, 79.93) |
66.09 | 0.342 | |
UA (°) | 70.56±1.88 (66.96, 74.17) |
66.86±1.69 (63.26, 70.48) |
70.46 | 0.151 |
Data presented as mean±SEM. SEM: Standard error of the mean, CF: Compressive force, SF: Shearing force, JRF: Joint reaction force, ESF: Erector spinae force, FA: Forearm, UA: Upper-arm, N: Newton, Nm: Newton-meter, (°): Degree, CI: Confidence interval, Coef: Coefficients, Inc.: Inclination, NL: No-load, L: Load
DISCUSSION
The present study was undertaken to determine the kinetic and kinematic responses on gradient walking while carrying a 30 kg load. It was observed from the t-test analysis responses of kinetic parameters such as compressive force, shearing force, torque, joint reaction force, and erector spine forces are likely to show a significant increase with increasing load. However, in kinematic analysis, only the angular changes of the neck and trunk showed significant changes with increment of load.
According to Wang et al., walking with a loaded carriage results in greater active, braking, impact, loading, and maximal breaking forces than walking without a load. Hip flexion and range of motion were considerably greater in kinematic characteristics when compared to walking off without a load.[13] Increment of force exertion during uphill walking due to activation of leg muscle and muscle stimulation of calves, glutes, and hamstrings. During uphill walking, the human body exerts more force than level walking due to pushing a mass of the body toward antigravity. While taking a 30 kg external load above that, gravity will always pull the external weight toward the ground with applied force in the long axis of the body.
To equilibrate, the push and pull of the body exert more compressive force compared to no-load conditions. The compressive force that affects the L5/S1 spinal disc is 3425 N.[14] In this study, the compressive force increases from 762.3 to 1967.7 N, almost 2.6 times higher than the no-load condition and 74.12% near the action limit. Based on the injury risk of compressive force, it may be suggested at 10° inclination at a walking speed of 3.5 kmph, the load should not exceed 30 kg for a long duration. At this inclination and speed, increment of load demands more compressive force acting on joints, and this exertion force alters with alteration of speed and inclination.
Elevation of thoracic load may alter respiratory frequency rate followed by respiratory muscles’ early fatigue. This leads to metabo-reflex of the lungs (mechanical restriction of lung function), alters metabolic cost, and increases oxygen consumption and vasoconstriction in the lower extremities, causing increased heart rate and blood pressure.[15]
The increase in impact pressure would change the kinematics of the lower limbs.[16] When a human walks in a particular direction, irrespective of gradient, both feet administer force in opposite directions. One foot exerts pressure on the ground in forward direction, whereas the other foot applies force in a backward direction at a perpendicular angle. The combination of exerted forces is called shear force. In uphill walking, the body needs to push more force to continue a gait cycle. The amount of shearing force that may cause injury on the L5/S1 joint of the spinal cord is 900 N.[17] Our results from the conducted study showed that exerting shearing force was 4.65 folds increased with load condition, and its almost 86% near to moderate injury risk. The amount of load at this speed and inclination should not be increased, as further it causes an increment of shearing force. The body might face musculoskeletal injury incidence, ligament injury due to extra force in parallel hitting the lower extremities.
Torque is the product of force and distance. It always acts on the line of action to the motion axis. When one walks uphill, torque continuously changes with a line of action and motion axis. In addition, another torque, that is, frictional torque from the surface, acts at the foot surface, opposing the gravitational force, which leads to generate some extra torque. Inclined walking with load causes more couple of torques over joint angular changes. As proposed by OSHA, the lumbosacral torque ≥ 163 Nm is considered hazardous.[18] The study results depict that the exerted torque is only 65.12 Nm while inclined walking with a 30 kg load. It is suggested that the risk of injury might be less at this speed and inclination, but it may be increased with increment of load, inclination, and speed accordingly.
Joint reaction forces can be defined as a force generated within a joint to equilibrate the force acting on the joints unless it increases the risk of injury. According to Bergmann et al.[19] and Layton et al.,[20] walking is the most strenuous activity, and walking incline average peak forces exerted from joints are 1800 N, and highest 3900 N. Hip joint reaction force ranges varied from 0.5 to 9 folds of body weight. A Harvard School of Medical article[21] stated that while walking across the ground level, the exertion of force on the knee is equivalent to 1.5 times a person’s body weight. Inclined walking needs extra force to push up a person’s body weight against gravitational force. Moreover, Kim et al. stated that the maximum joint reaction force exerted by the ankle while walking is 8.72% of body weight. The maximum joint reaction force of a human body is 6.4 times the body weight.[22] The study result showed that the exerted net joint reaction forces of the body were 2.83 times higher than in no-load conditions. It is suggested from the entire result that injury risk might be higher at 10-degree inclination walking with a 30 kg load at 3.5 kmph speed. The rate of injury risk will be much higher with alteration in inclination, speed, and load.
The importance of erector spine force is required to move the vertebral column with gait rhythm. Bilaterally, the contraction muscle extends the spine during walking. When walking inclined, the centre of gravity will always be changing, and the body exerts an opposite and equal force to keep the spine erect. While walking inclined with external load conditions, the body needs to exert extra force to erect the spine. Literature states that the erector spine force of the body is 170 N, 100 N, and 600 N as standing, 5° extension, and 30° flexion of the body, respectively. The maximum exerted forces during spine erection and torque balancing is 1513 N.[23] This study’s results showed it increased 4.06 folds as compared to no-load conditions at 10° uphill walking while carrying a 30 kg load at a 3.5 kmph pace and 83.82% near hazardous conditions. It might cause hazardous musculoskeletal injury.
A study by Saeki et al., stated that the optimum angle of joints and production of force has been correlated to each other. The muscle lengthens by an increase in muscle length (joint angle) that changes the force production.[24]
When lifting loads more than 10 kg, the joint angle reportedly flexes significantly, and the trunk, ankle, and knee angles are changed, as per Attwells et al.[25] and Murray et al.[26] In this study, results showed that the movement of trunk and neck joint angles significantly differs from the no-load condition. Still, upper arm, forearm, thigh, and leg joint angles did not differ significantly. In light of the findings, 30 kg compact load carriage is more demanding on trunk movement and neck movement to counterbalance the gravitational force. As measured to the horizontal axis, neck and trunk angle almost two times significant decrease as compared to no-load condition. Other kinematic changes also occur in the thigh, leg, and arm but are not statistically approved.
There are two other forces simultaneously more active with exertion joint forces at uphill walking compared to level walking static and kinetic frictional forces. In general, friction is described as a contact force that prevents surfaces from sliding against one another.
Static friction always pushes in the moving direction when a person walks and prevents the foot from slipping, which causes forward motion. However, the kinetic friction always pushes downwards. To counterbalance these frictional forces, the human body needs to push more against these frictional forces, resulting in an increment of compressive, shearing, torque, joint reaction force, and erector spine forces with an incline position added with the load.
Walking uphill, the body’s mechanism is such that normal force (perpendicular) and frictional force (parallel) act on two axes of ground. However, the weight of the human body acts along with both axes (X, Y). The perpendicular force (N) is similar to the perpendicular weight magnitude (Y-axis) due to no motion in this direction. Still, the parallel frictional force is higher than the weight magnitude (X-axis) due to uphill walking. To equilibrate, the body needs to exert more force. While carrying an external load, the exerted forces were more, due to which the body needs to push body weight along with additional load. However, the results of this study can be used to calculate the recommended load carriage limit at different gradients.
CONCLUSION
The present study concluded that with increasing load, the body’s biomechanical responses were significantly increased as compared to the no-load condition at 10° positive gradient, 3.5 kmph speed. Based on the study results, it is suggested that compressive force 8.6, shearing force 15.3, torque 13.5, joint reaction force 9.33, and erector spinae force 13.52 folds increase, whereas angular changes of neck and trunk 5.92 and 4.16-folds decrease respect to horizontal axes, respectively, as the increment of load at 10° uphill gradient at the specified speed of walking without considering terrain, external environment factor and altitude factors. The exerted forces are very close to injury risk, namely compressive force 74.12%, shearing force 86%, joint reaction force almost 75%, and erector spinae spine force 83.82%; only changes of torque not much closer to injury risk. Based on the obtained findings of the present study, it is suggested at 10° inclination with a walking speed of 3.5 kmph, the load should not exceed 30 kg for a long duration. Beyond this limit might cause severe musculoskeletal injuries. The combination of weight and gradient data can be used to recommend load carriage limits at different gradient levels in future studies.
Limitations
The present study is restricted to an uphill gradient, one walking speed, a 30 kg load, and controlled laboratory conditions only. The recommendation of further study on heavy loads, real fields, varying speeds, varying gradients, and other biomechanical factors for different terrains is required.
Acknowledgment and author’s contributions
Participants’ contributions are appreciated; the authors would like to appreciate their effort for contribution in this study. MP was involved in experiment design, overall technical and administrative guidance, and supervision. VS was involved in data collection, interpretation, and manuscript preparation. SDK was involved in manuscript writing, data analysis, interpretation, and statistical analysis. All authors read and approved the final manuscript.
Ethical approval
This study was approved by the Institutional Ethical Committee. (Ref no: IEC/DIPAS/dated 12.01.21).
Declaration of patient consent
Patient’s consent not required as there are no patients in this study.
Conflicts of interest
There are no conflicts of interest.
Use of artificial intelligence (AI)-assisted technology for manuscript preparation
The authors confirm that there was no use of artificial intelligence (AI)-assisted technology for assisting in the writing or editing of the manuscript and no images were manipulated using AI.
Financial support and sponsorship
This study was financially supported by the Defence Research and Development Organization, Ministry of Defence, Government of India.
References
- Speed marching in military boots: The walk-to-run transition speed and vertical ground reaction forces. Int J Sports Exerc Med. 2022;8:214.
- [CrossRef] [Google Scholar]
- Prevention and treatment of exercise related leg pain in young soldiers; a review of the literature and current practice in the Dutch Armed Forces. J R Army Med Corps. 2017;163:94-103.
- [CrossRef] [PubMed] [Google Scholar]
- Why forefoot striking in minimal shoes might positively change the course of running injuries. J Sport Health Sci. 2017;6:154-61.
- [CrossRef] [PubMed] [Google Scholar]
- Biomechanics of military load carriage and resulting musculoskeletal injury: A review. J Orthop Orthop Surg. 2020;1:6-11.
- [CrossRef] [Google Scholar]
- The effect of carried loads on the walking patterns of men and women. Ergonomics. 1986;29:1191-202.
- [CrossRef] [PubMed] [Google Scholar]
- Backpack vs. front-back pack: Differential effects of load on walking posture. Med Sci Sports Exerc. 1994;26:S140.
- [CrossRef] [Google Scholar]
- The effects of backpack weight on the biomechanics of load carriage. Army Res Inst Environ Med Natick Ma Mil Performancediv. 2020;1:1-62.
- [Google Scholar]
- Load carriage using packs: A review of physiological, biomechanical and medical aspects. Appl Ergon. 1996;27:207-16.
- [CrossRef] [PubMed] [Google Scholar]
- Soldier load carriage: Historical, physiological, biomechanical, and medical aspects. Mil Med. 2004;169:45-56.
- [CrossRef] [PubMed] [Google Scholar]
- Optimum load for carriage by Indian soldiers on different uphill gradients at specified walking speed. Int J Ind Ergon. 2014;44:260-5.
- [CrossRef] [Google Scholar]
- Determinants of load carrying ability. Appl Ergon. 1988;19:111-21.
- [CrossRef] [PubMed] [Google Scholar]
- Cardio-respiratory and metabolic changes during continuous uphill-downhill load carriage task. 2015. Report published in HWWE-2013. :3-10. Available from: https://inet.vidyasagar.ac.in:8080/jspui/handle/123456789/193 [Last accessed on 2023 Nov 11]
- [Google Scholar]
- Effect of load carriage lifestyle on kinematics and kinetics of gait. Appl Bionics Biomech. 2023;2023:8022635.
- [CrossRef] [PubMed] [Google Scholar]
- Clinical biomechanics of the spine In: Clinical Biomechanics of the Spine. United States: Lippincott; 1990.
- [Google Scholar]
- The physiology and biomechanics of load carriage performance. Mil Med. 2019;184:e83-90.
- [CrossRef] [PubMed] [Google Scholar]
- Effects of load carriage and footwear on lower extremity kinetics and kinematics during overground walking. Gait Posture. 2016;50:207-11.
- [CrossRef] [PubMed] [Google Scholar]
- Biomechanical analysis and assessment of lumbar stress during load lifting using a dynamic 19-segment human model. Ergonomics. 1989;32:93-112.
- [CrossRef] [PubMed] [Google Scholar]
- Realistic loads for testing hip implants. Biomed Mater Eng. 2010;20:65-75.
- [CrossRef] [PubMed] [Google Scholar]
- Characteristics of hip joint reaction forces during a range of activities. Med Eng Phys. 2022;108:103894.
- [CrossRef] [PubMed] [Google Scholar]
- Why weight matters when it comes to joint pain. 2016. Harvard Health. Available from: https://www.health.harvard.edu/pain/why-weight-matters-when-it-comes-to-joint-pain#:~:text=When%20you%20walk%20across%20level,his%20knees%20with%20each%20step [Last accessed on 2023 Nov 11]
- [Google Scholar]
- Joint moments and contact forces in the foot during walking. J Biomech. 2018;74:79-85.
- [CrossRef] [PubMed] [Google Scholar]
- Harvard natural sciences lecture demonstrations. 2023. Available from: https://sciencedemonstrations.fas.harvard.edu/search/site/balancing%20torque [Last accessed on 2023 Nov 11]
- [Google Scholar]
- Optimum angle of force production temporarily changes due to growth in male adolescence. Children (Basel). 2021;8:20.
- [CrossRef] [PubMed] [Google Scholar]
- Influence of carrying heavy loads on soldiers' posture, movements and gait. Ergonomics. 2006;49:1527-37.
- [CrossRef] [PubMed] [Google Scholar]
- Comparison of free and fast speed walking patterns of normal men. Am J Phys Med. 1966;45:8-23.
- [CrossRef] [PubMed] [Google Scholar]