Translate this page into:
Diallyl disulfide regulates purine metabolism and their metabolites in diabetes mellitus
*Corresponding author: Dr. Prashanthkumar Goudappala, Assistant Professor, Department of Biochemistry, World College of Medical Sciences and Research And Hospital, Jhajjar-124 103, Haryana, India. prashanth13jan@gmail.com
-
Received: ,
Accepted: ,
How to cite this article: Goudappala P, Gowda CV, Kashinath RT. Diallyl disulfide regulates purine metabolism and their metabolites in diabetes mellitus. Indian J Physiol Pharmacol 2021;65(1):28-34.
Abstract
Objectives:
The garlic and its principle sulfur compound, diallyl disulfide (DADS), have been claimed to have hypoglycemic activity and are helpful in achieving glycemic control in diabetes mellitus. The exact uric acid mechanism about the DADS controls on glucose level in diabetes mellitus is not clearly established though it is known that DADS does influence insulin action probably by improving insulin half-life. A study was undertaken to examine the role of DADS on purine metabolism and their metabolites in the diabetic condition and the probable connection of uric acid, xanthine oxidase and adenosine deaminase (ADA) enzymes in rat model.
Materials and Methods:
The induction of diabetes was done by alloxan and diabetic rats were treated with DADS for 30 days and compared with the standard hypoglycemic drug metformin. Purine metabolites alteration was measured by the activities of ADA, xanthine oxidase, and uric acid in the diabetes induced rats.
Results:
DADS which exhibits its strong action on glucose utilization in the liver tissues by regulating the purine metabolites in diabetic condition showing favorable changes in the levels of xanthine oxidase, ADA, and uric acid levels.
Conclusion:
This establish the possible effect of DADS on purine metabolites in alloxan diabetic liver tissues and to associate this action of DADS with metformin which is a common drug used for treating type-2 diabetes mellitus. DADS can be used as a therapeutic drug for glycemic control in diabetes mellitus.
Keywords
Adenosine deaminase
Diallyl disulfide
Diabetes
Metformin
Xanthine oxidase
INTRODUCTION
Diabetes mellitus is a chronic metabolic disorder due to either deficiency of insulin or subnormal function of insulin.[1] Glucose metabolism is primarily affected in diabetes mellitus as insulin is known to influence it particularly.[2] Patients with uncontrolled blood glucose levels are particularly at high risk and consistent hyperglycemia plays a significant role in the pathogenesis of diabetes induced complications.[3]
The researches have exposed that high uric acid levels controls the oxidative stress, inflammation and enzymes related with lipid and glucose metabolism, and signifying a mechanism for the weakening of metabolic homeostasis. The uric acid level depends on the equilibrium between the purine production, absorption, and excretion. Uric acid is produced in the adipose tissue, muscle, and liver and is principally expelled over the urinary tract. A high level of uric acid has frequently been connected with the diabetes mellitus risk and its metabolism is also closely connected to fructose, glucose levels, and obesity.[4,5] Likewise, xanthine oxidase, a metalloflavoenzyme catalyzes hypoxanthine oxidation to xanthine and then to uric acid. Apart from its activity in uric acid formation, xanthine oxidase also produces oxidants, which are key performers in the development of Type II diabetes mellitus [6], no research has found the uric acid correlation between the effect of serum xanthine oxidase and the risk of developing diabetes mellitus.
Adenosine acts directly on tissues to elicit insulin effect through numerous processes such as augmented glucose transport, pyruvate dehydrogenase activity, lipid synthesis, cyclic nucleotide phosphodiesterase effect, and leucine oxidation.[7,8] Adenosine levels are, in turn, controlled by serum ADA and it has been demonstrated that adenosine through ADA action plays a significant role in the inflection of glucose metabolism in various tissues.[9]
The World Health Organization has also suggested the assessment of plants effectiveness in controlling widespread increase of diabetes mellitus as we lack in safe modern drugs.[10] This has resulted in the augmenting demand for investigation on natural anti-diabetic products which yields no or minimal side effects. Allium group of plants was evidenced to have beneficial possessions in diabetes mellitus.[11] Diallyl disulfide (DADS or 4, 5-dithia-1, 7-octadiene), an important phytocompound from garlic possess antitumor and immunomodulatory functions have attracted attention of researchers.[12] Earlier studies reported that DADS have antiulcer, antioxidant activities and also DADS affects the insulin activity in alloxan-induced diabetic rats.[13]
Previously, we studied the stimulatory effect of DADS on glycogen breakdown in alloxan induced diabetic liver.[14] Another study suggested that increase of hexokinase and glucose-6-phosphate dehydrogenase (G6PD) activities can be due to the influence of glucose utilization by DADS.[15] DADS significantly showed protective role of DADS in hyperglycemia mediated oxidative damage and its antioxidant potential.[16] DADS suppressed gluconeogenesis cycle and precursors for gluconeogenesis were considerably decreased.[17] Earlier reports supports the current study to elucidate the effects of DADS on diabetes mellitus for the extensive development and use of garlic extracts. Hence, a study was commenced to establish the possible effect of DADS on purine metabolites alloxan diabetic liver tissues and to associate this action of DADS with metformin (dimethylbiguric acidnide), which is a common drug used for treating type-2 diabetes mellitus.
MATERIALS AND METHODS
Chemicals
The chemicals such as DADS, metformin and alloxan were procured from Sigma-Aldrich Chemicals (St. Louis, U.S.A.) and other organic solvents and chemicals used were of analytical grade procured from Thermo fisher Scientific, India. The standards for xanthine oxidase and adenosine deaminase (ADA) obtained from Randox Laboratories.
Animals
The male Wistar albino rats having 150–200 g weight was taken for the present studies. They were housed in clean polypropylene cages and maintained under standard laboratory condition at a temperature 22 ± 2°C and 12 h alternating light-dark cycle. They were permitted unrestricted access to standard pellet diet (Hindustan Lever, Kolkata, India) and water ad libitum. The experiments were conducted as per the stipulations of CPCSEA under the supervision of Institutional Animal Ethics Committee (Permission No: BMCH/IAEC/04 Biochem/2015).
Diabetes induction
Induction of diabetes was done using freshly made solution of Alloxan monohydrate liquified in normal saline in 18 h fasted rats as a single dose (150 mg/kg; i.p.). Hyperglycemia was being confirmed by elevated glucose level in plasma resolute at 48 h after injection and the animals tested for glucose positive for 3 consecutive days. Rats with blood-glucose levels beyond 250 mg/dL were measured as diabetic and used for the study.
Experimental design
The animals were categorized into four groups having six animals in every group. Group I rats were administered with normal saline (1 ml/kg.b.wt) and Group II rats were intraperitoneally injected with alloxan (150 mg/kg/day) as a single dose for inducing diabetes (Caraway and Hald, 1963). The Group III animals were given alloxan and treated with metformin (150 mg/kg/day) orally for 30 days. Finally, fourth group of animals was administered with alloxan and treated with DADS (100 mg/kg/days) orally for 30 days.
After 30 days, the rats were deprived of diet whole night. The rats were euthanized in chloroform vapor. Using a sterile scalpel blade, the jugular vein was wide-open and cut, and the animals were bled into specimen bottles. Blood samples were shifted to sanitized centrifuge tubes and kept at room temperature for clotting. The centrifugation of the blood samples was done at 1500 rpm for 10 min and kept at –20°C until the investigation was carried out. The blood glucose was assessed by orthotoluidine method and dignified using UV-Visible spectrophotometer at 540 nm. Liver was dissected out, rinsed in ice cold saline and weight was taken. Liver tissue homogenate was made using PBS and preserved at –4°C until further assay. The total protein levels are estimated using Bradford assay and this protein concentration is used for measuring the activities of enzymes carried out in this study.
Estimation of uric acid
Uric acid was assessed conferring to the method of Caraway (1963). Diluted tungstic acid (5.4 ml) was added to the plasma sample (0.6 ml), the mixture was centrifuged at 3500× g using a centrifuge. About 3 mL of supernatant, water (as blank), and standard were taken in test tube, then sodium carbonate (0.6 ml) and phosphotungstic acid reagent (0.6 ml) purchased from SRL laboratories, India. Were added, mixed and kept in a water bath for 10 min at 25°C. The blue color formed was read at 700 nm. The levels of uric were measured as mg/dl.[18]
Estimation of xanthine oxidase
The xanthine oxidase activity was examined by the method of Hashimoto (1974). The chemicals required for this enzyme estimation were purchased from Sigma-Aldrich Company, US. In ice-cold sucrose (0.25 M; 9 volumes), the liver tissue was homogenized. The sonicated homogenate was kept at 4°C (10,000 g for 20 min), and the supernatant was dialyzed in contrast to 100 volumes of the same solution for 24 h at 4°C. In the test tubes 3.0 ml of incubation mixture contains 150 μM phosphate buffer, 0.2 μM xanthine, 0.3 μM potassium oxidase, and 0.2 ml of tissue homogenate. The tubes were kept for 30 min at 30°C. 0.1 ml of 100% of TCA was added and mixed well. The content centrifuged at 10000xg for 15 min and supernatant was analyzed for xanthine oxidase activity. The test and standards were read against the blank at 292 nm. One unit (U) of this enzyme is demarcated as the uric acid quantity of enzyme creating 1 μmol uric acid/min. The effect of xanthine oxidase was expressed as U/g protein.[19]
Assay of ADA
The serum ADA level was measured using a spectrophotometer depending on the method of Oosthuizen et al. (1993). The chemicals required for this enzyme estimation were purchased from Sigma-Aldrich Company, US. ADA hydrolyses adenosine to ammonia and inosine. In an alkaline medium, ammonia then act in response with a phenol and hypochlorite to create a colored blue indophenol complex, by means of sodium nitroprusside as the catalyst. The blue indophenol complex degree is directly related to the effect of ADA in the sample. The absorbance was noted against water at 635 nm by means of a spectrophotometer. One unit of ADA is described as the measure of enzyme needed to discharge 1 mmol of ammonia per minute from adenosine at standard analysis conditions.[20]
Statistical analysis
Data acquired from the experiments (n = 6) are measured as mean ± SD. These data were exposed to one-way analysis of variance for statistical analysis, monitored by Student’s t-test. A level of p < 0.05, p < 0.01, and p < 0.001 was taken as significant. The statistical analysis was performed using the SPSS statistical package (version 22.0).
RESULTS
The effect of DADS and metformin on xanthine oxidase activities in alloxan-induced diabetic rats is given in [Figure 1]. It is evident from the data that activity of xanthine oxidase was significantly (p < 0.001) elevated in the diabetic group as compared to normal rats whereas the activities are significantly (p < 0.05) reduced in DADS and metformin treated rats as compared to diabetic group. There was no significant difference between metformin and DADS treated diabetes mellitus administered rats in the level of xanthine oxidase. Effect of DADS and metformin on liver tissue protein levels in diabetes mellitus induced rats has been given in [Table 1].
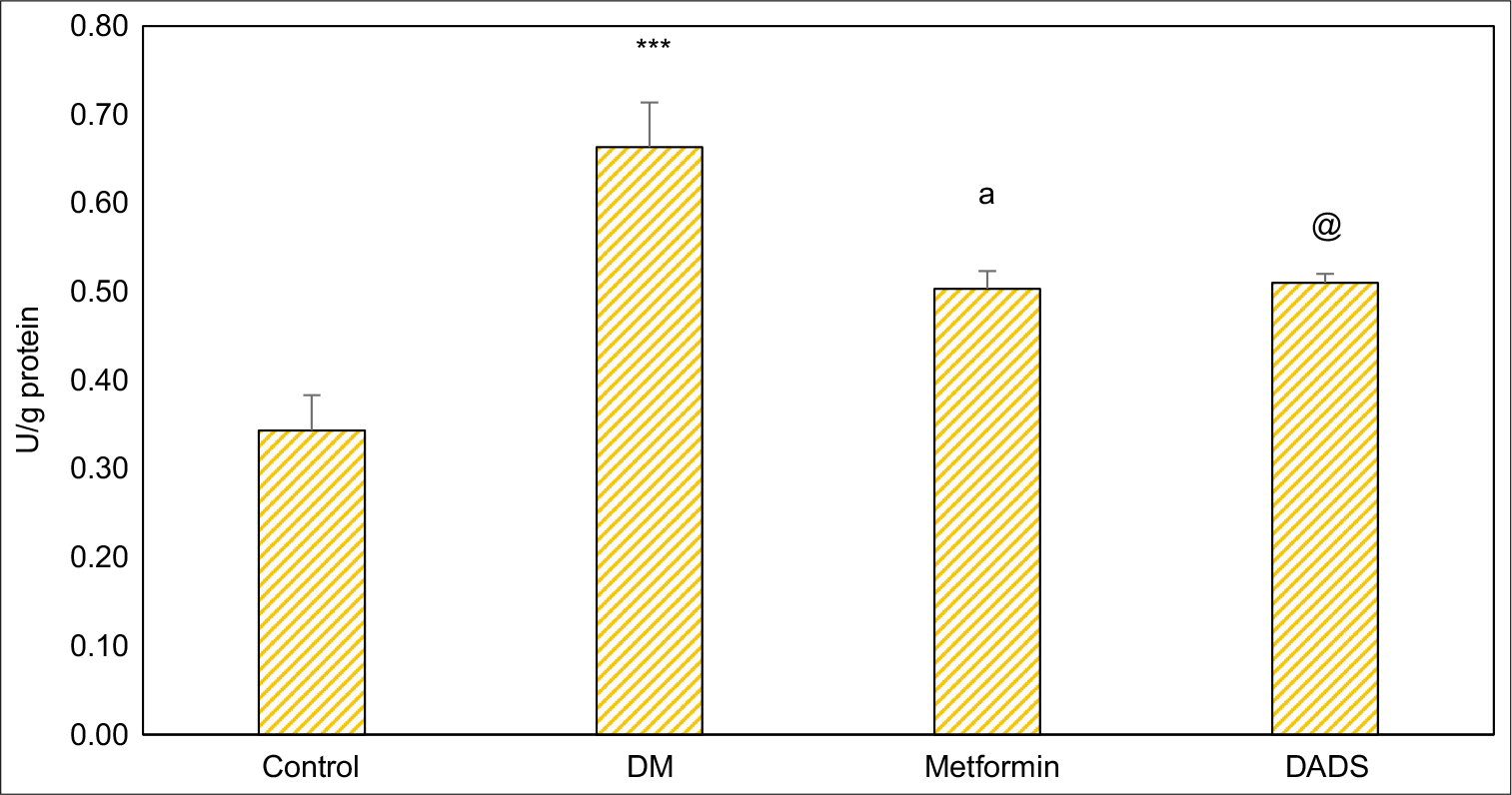
- Effect of diallyl disulfide and metformin on xanthine oxidase activities in diabetes mellitus induced rats. Results are expressed as mean ± SD (n = 6); ***P <0.001 as compared to normal control group; aP <0.05 as compared to diabetes mellitus (DM) control; @P <0.05 as compared to DM control.
Groups | Protein (mg/g tissue) |
---|---|
Control | 142.3±7.4 |
DM | 157.8±6.9*** |
Metformin | 146.5±5.3a |
DADS | 149.3±7.1@ |
Results are expressed as mean±SD (n=6); ***P<0.001 as compared to normal control group; a P<0.05 as compared to DM group; @P<0.05 as compared to DM group. DADS: diallyl disulfide, DM: Diabetes mellitus
The expression of ADA was increased in diabetic rats in contrast to normal control group. We noted that the DADS decreased significantly (p < 0.05) the ADA activity similar to that of metformin. The effect of DADS and metformin on the ADA activities in diabetes mellitus induced rats is depicted in [Figure 2].
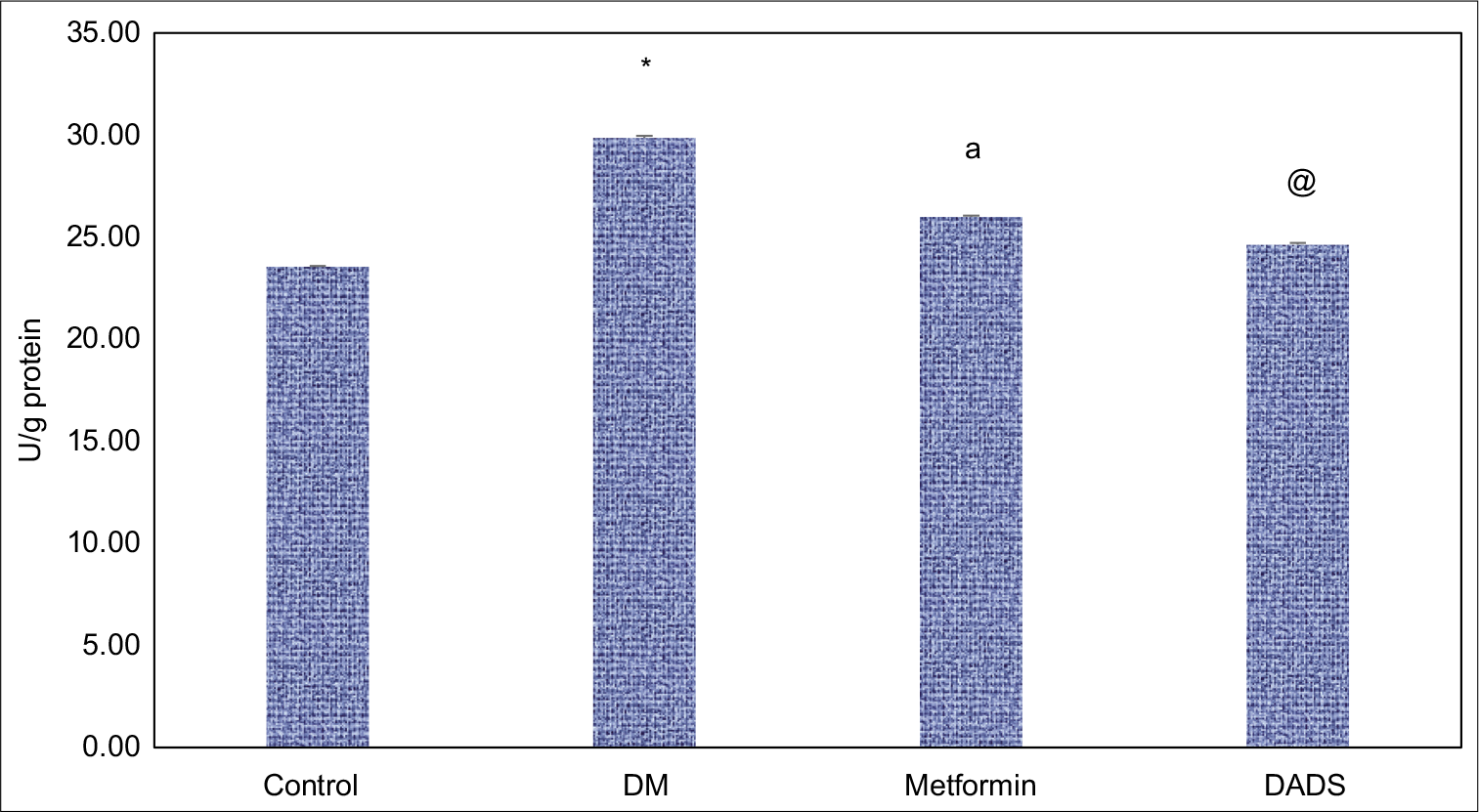
- Effect of diallyl disulfide and metformin on adenosine deaminase activities in diabetes mellitus induced rats. Results are expressed as mean ± SD (n = 6); *P <0.05 as compared to normal control group; aP <0.05 as compared to diabetes mellitus (DM) group; @P <0.05 as compared to DM group.
The uric acid levels were (p < 0.05) significantly upregulated in diabetic rats in contrast to normal control group. DADS treatment ameliorates (p < 0.05) similar to the uric acid level in diabetic rats significantly in comparison with diabetic untreated rats. There was no significant difference between metformin and DADS treated diabetes mellitus administered rats in the level of uric acid which is shown in [Figure 3].
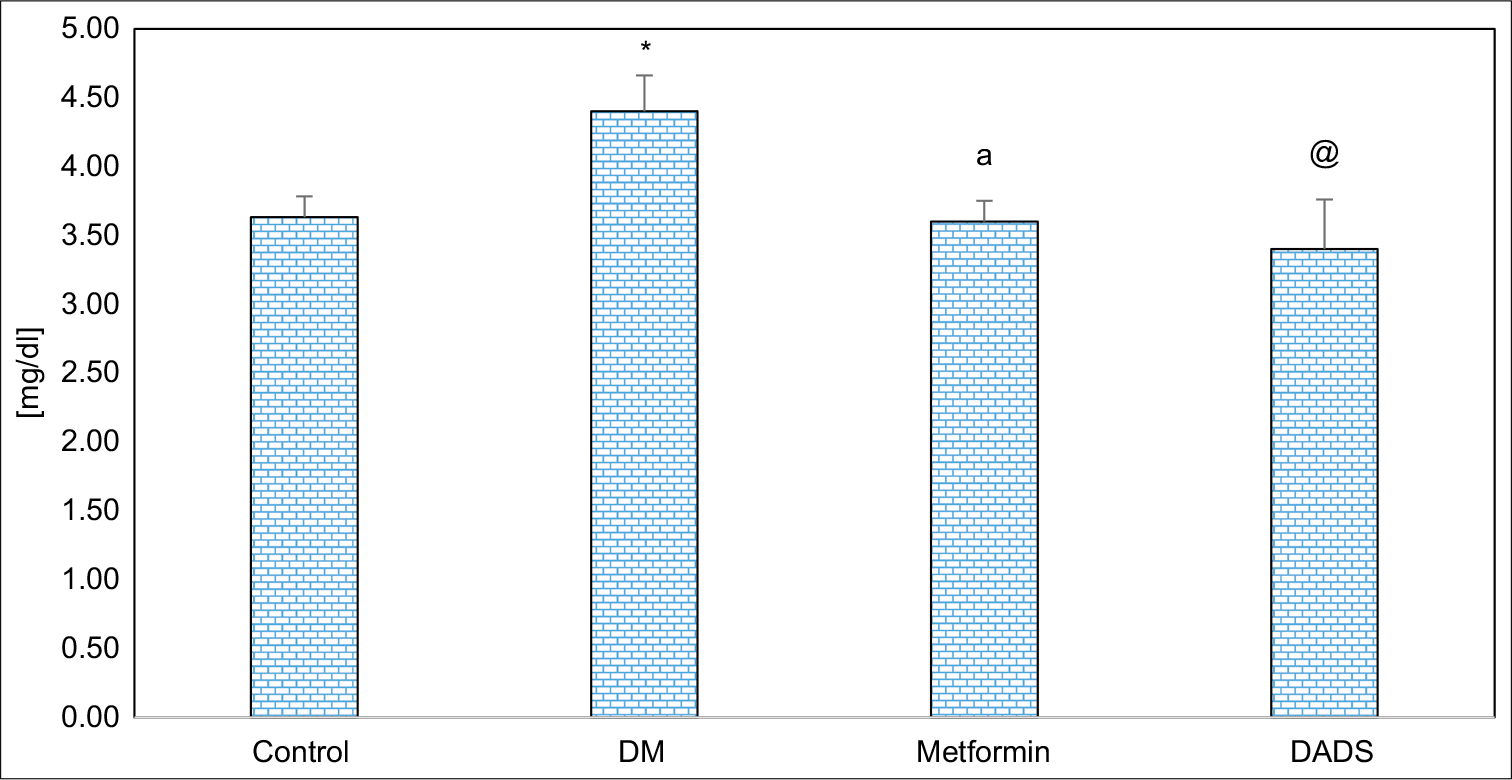
- Effect of diallyl disulfide and metformin on uric acid levels in diabetes mellitus induced rats. Results are expressed as mean ± SD (n = 6); *P <0.05 as compared to normal control group; aP <0.05 as compared to diabetes mellitus (DM) group; @P <0.05 as compared to DM group.
DISCUSSION
The diabetes treatment goals are correlated to control blood glucose, lipids, and blood pressure to diminish the risk of long-term concerns associated with diabetes. The difficulties of diabetes which contains the abnormalities formed in lipids and proteins are the main etiologic aspects. Rise in hepatic glucose production is the main cause of fasting hyperglycemia. In purine catabolism, their monophosphate forms are converted to inosine and uric acid; purine nucleoside phosphorylase converts them to hypoxanthine and guanine, respectively. Xanthine oxidase and guanine deaminase, convert them to xanthine. Finally, xanthine is again oxidized by xanthine oxidase to uric acid. Purine metabolism is important in diabetes mellitus because purine levels can be useful for monitoring the progression of diabetes and treatment evaluation. Purine metabolites can be a marker to understand the progression of diabetes. [21] Blood levels of uric acid are well regulated in healthy subjects but when hyperuricemia occurs; its chronicity has been associated with diabetes mellitus. The levels of purine metabolites were reported in patients with diabetes indicating an increased oxidative stress in this metabolic condition.[22,23] Previously reports of this experiment showed urine metabolites such as uric acid and other intermediate products of purine are increased in diabetes.
ADA plays a key role in lymphocyte proliferation and differentiation and shows its maximum effect in T-lymphocytes. In the current study, we observed an important increase in the levels of ADA in diabetic group in contrast to controls. The high ADA effect can be because of abnormal T-lymphocyte proliferation that might point towards a mechanism that includes its release into circulation.[24,25] Earlier, ADA has been described to be an indicator for insulin function.[26] ADA is recommended to be a significant enzyme for moderating the bioactivity of insulin. Comparable to our study results, Niraula et al. (2018) also established an important escalation in serum ADA effect in diabetes mellitus with increase in HbA1c levels, recommends a significant role in predicting the immunological and glycemic status in diabetic controls.[26]
The main physiologic purpose of xanthine oxidase is in purine catabolism, where it oxidizes hypoxanthine to xanthine and xanthine to uric acid. Although both the oxidase and dehydrogenase forms of the enzyme can decrease molecular oxygen to hydrogen peroxide and superoxide, only xanthine oxidoreductase can reduce NAD and form NADH.[27] The enzyme is supposed to be one of the key enzymes in cellular injury brought by O2 and connected active oxygen species. Similar to the present study result, the augmented xanthine oxidase activity witnessed in later stages of diabetes that can result in the enhanced oxidative stress in the kidney, heart, and brain, causing secondary organ damage related with the disease.[28]
Uric acid is measured as one of non-enzymatic antioxidant, but augmented production of uric acid means increased free-radical production due to activation of the xanthine oxidase enzyme system.[29] In our research, uric acid levels were augmented in diabetic rats, which is because of metabolic disturbance in diabetes replicated in higher effects of lipid peroxidation, xanthine oxidase, and augmented cholesterol and triglyceride. Furthermore, protein glycation in diabetes results in muscle wasting and augmented discharge of purine, as well as in effects of xanthine oxidase. Serum uric acid levels elevates with rising serum insulin levels in diabetic patients. Apart from hypoglycemic agents and insulin, many herbal preparations have been tried to expand glucose utilization in diabetes mellitus.[30] In general, allium group of plants and garlic products in particular were demonstrated to have advantageous effects in diabetes mellitus.[31]
Our study showed that the relationship between the effect of elevated xanthine oxidase and the high risk of incidence of diabetes mellitus is significant. This is consistent with findings from studies done by Pfister et al. (2011).[32] We noted that the test compound DADS lowered the expression of uric acid, xanthine oxidase, and ADA. Although it is known that garlic or one of its active principles, DADS as well as other disulfides may influence insulin action or increase insulin’s half-life, the actual mechanism of action was unclear.[32] Through the present study, it is clear that DADS influence on glucose utilization in diabetic controls. It is evident that the glucose utilization is significantly elevated in DADS exposed diabetic erythrocytes, further establishing the positive influence of DADS on glucose utilization in diabetic erythrocytes. DADS may not interfere with the activities of glycolytic enzymes. This may be explained that DADS might have undergone sufficient reduction to produce its thiol derivatives and these thiols might have interacted with sulfhydryl enzymes of glycolytic pathway thus favoring their actions by keeping these enzymes in their thiol forms. This study indicates that the active principles of garlic (diallyl disulfide) are hypoglycemic and increases the glucose utilization. The capacity of therapeutic compounds comprising medicinal plants to restore homeostasis in hyperglycemic state is an index of their antidiabetic effect and relevance. DADS has been claimed to have hypoglycemic effect, thus the present study signifies that DADS is advantageous in attaining glycemic control in diabetes mellitus. Principally, any protein or enzyme having accessible thiol groups which are vital for its effect is capable of yielding itself to variations in cellular thiol-disulfide ratio thus creating such enzymes or proteins for easy modulation. This may be reason for the favorable regulation of purine metabolites by DADS.
CONCLUSION
It can be concluded that DADS lowered the levels of uric acid, xanthine oxidase, and ADA in the diabetic control. It has a role in glucose turnover and can hold potentials for the balance of glucagon and insulin to maintain blood glucose. Our study proved that DADS therapy altered an inverse association between purine metabolites with incident T2D risk. However, a detailed study with systematic clinical approach can throw further light on DADS use in diabetes mellitus.
Declaration of patient consent
Patient’s consent not required as patients identity is not disclosed or compromised.
Financial support and sponsorship
Nil.
Conflicts of interest
There are no conflicts of interest.
References
- Hyperglycemia-driven neuroinflammation compromises BBB leading to memory loss in both diabetes mellitus (DM) Type 1 and Type 2 mouse models. Mol Neurobiol. 2019;56:1883-96.
- [CrossRef] [PubMed] [Google Scholar]
- Global aetiology and epidemiology of Type 2 diabetes mellitus and its complications. Nat Rev Endocrinol. 2018;14:88-98.
- [CrossRef] [PubMed] [Google Scholar]
- Periodontal complications of hyperglycemia/diabetes mellitus: Epidemiologic complexity and clinical challenge. Periodontology. 2018;78:59-97.
- [CrossRef] [PubMed] [Google Scholar]
- Fructose metabolism and metabolic disease. J Clin Invest. 2018;128:545-55.
- [CrossRef] [PubMed] [Google Scholar]
- The serum uric acid concentration is not causally linked to diabetic nephropathy in Type 1 diabetes. Kidney Int. 2017;91:1178-85.
- [CrossRef] [PubMed] [Google Scholar]
- Hyperuricemia and cardiovascular risk In: High Blood Press Cardiovasc Prev. Vol 21. 2014. p. :235-42.
- [CrossRef] [PubMed] [Google Scholar]
- Antioxidant effects and mechanisms of medicinal plants and their bioactive compounds for the prevention and treatment of Type 2 diabetes. Oxid Med Cell Longev. 2020;2020:1356893.
- [CrossRef] [PubMed] [Google Scholar]
- Relationship between serum adenosine deaminase levels and liver histology in autoimmune hepatitis. World J Gastroenterol. 2017;23:3876-82.
- [CrossRef] [PubMed] [Google Scholar]
- A study on the correlation of adenosine deaminase and glycated haemoglobin in the patients of Type 2 diabetes mellitus. Int J Med Biomed Stud. 2019;3:55.
- [CrossRef] [Google Scholar]
- Use of complementary and alternative medicine by patients with breast cancer: Observations from a health-care survey. Support Care Cancer. 2004;12:789-96.
- [CrossRef] [PubMed] [Google Scholar]
- Effect of diallyl disulphide on diabetes induced dyslipidemia in male albino rats. J Clin Diagn Res. 2015;9:BF01-3.
- [CrossRef] [PubMed] [Google Scholar]
- Transcriptomic insights into the allelopathic effects of the garlic allelochemical diallyl disulfide on tomato roots. Sci Rep. 2016;6:38902.
- [CrossRef] [PubMed] [Google Scholar]
- Effect of diallyl disulphide on glucose utilization in isolated alloxan diabetic liver. Biomed Res. 2018;29:3207-12.
- [CrossRef] [Google Scholar]
- Influence of diallyldisulphide on glycogen breakdown in alloxan diabetic liver. IOSR J Dent Med Sci. 2017;16:30-2.
- [CrossRef] [Google Scholar]
- Diallyl disulfide improves the liver antioxidant potential in diabetes-mediated oxidative damage in rats. Drug Invent Today. 2019;11:22-31.
- [CrossRef] [Google Scholar]
- Influence of diallyl disulphide on hepatic gluconeogenesis suppression by CREB binding protein phosphorylation. Int J Res Pharm Sci. 2019;10:1327-31.
- [CrossRef] [Google Scholar]
- Effect of diallyl disulphide (DADS) on gluconeogenesis: A study in isolated alloxan induced diabetic liver. Pharmacogn J. 2019;11:32.
- [CrossRef] [Google Scholar]
- Uric acid In: Standard Methods of Clinical Chemistry. Vol 1. Cambridge: Academic Press; 1963. p. :239-47.
- [CrossRef] [Google Scholar]
- A new spectrophotometric assay method of xanthine oxidase in crude tissue homogenate. Anal Biochem. 1974;62:426-35.
- [CrossRef] [Google Scholar]
- Kinetic determination of serum adenosine deaminase. Clin Chem. 1993;39:2182-5.
- [CrossRef] [PubMed] [Google Scholar]
- Moonlighting adenosine deaminase: A target protein for drug development. Med Res Rev. 2015;35:85-125.
- [CrossRef] [PubMed] [Google Scholar]
- Extracellular adenosine-mediated modulation of regulatory T cells. Front Immunol. 2014;5:304.
- [CrossRef] [PubMed] [Google Scholar]
- Adenosine deaminase as marker of insulin resistance. Int J Res Med Sci. 2016;4:2972-9.
- [CrossRef] [Google Scholar]
- Evaluation of ADA, IL-6 and TNF-alpha level in Type 2 diabetes mellitus: With-and without hypoglycemic drugs. J Natl Sci Res. 2015;5:52.
- [Google Scholar]
- Adenosine deaminase activity in Type 2 diabetes mellitus: Does it have any role? BMC Endocr Disord. 2018;18:58.
- [CrossRef] [PubMed] [Google Scholar]
- Nitrite reductase activity of rat and human xanthine oxidase, xanthine dehydrogenase, and aldehyde oxidase: Evaluation of their contribution to No formation in vivo. Biochemistry. 2015;54:685-710.
- [CrossRef] [PubMed] [Google Scholar]
- Purine metabolites can indicate diabetes progression. Arch Physiol Biochem. 2019;1:1-5.
- [CrossRef] [PubMed] [Google Scholar]
- Prevention of protein glycation by natural compounds. Molecules. 2015;20:3309-34.
- [CrossRef] [PubMed] [Google Scholar]
- Current understanding of metformin effect on the control of hyperglycemia in diabetes. J Endocrinol. 2016;228:R97-106.
- [CrossRef] [PubMed] [Google Scholar]
- A mendelian randomization study of circulating uric acid and Type 2 diabetes. Diabetes. 2015;64:3028-36.
- [CrossRef] [PubMed] [Google Scholar]
- Osteocalcin is not associated with the risk of Type 2 diabetes: Findings from the EPIC-NL study. PLoS One. 2015;10:e0138693.
- [CrossRef] [PubMed] [Google Scholar]
- No evidence for a causal link between uric acid and Type 2 diabetes: A Mendelian randomisation approach. Diabetologia. 2011;54:2561-9.
- [CrossRef] [PubMed] [Google Scholar]