Translate this page into:
Differential strain sensitivity to low-dose anxiolytic diazepam in anxiety-related behaviour in male Wistar and Wistar-Kyoto, a rat model of endogenous depression
*Corresponding author: Monika Sadananda, Department of Biosciences, Mangalore University, Mangalagangotri - 574 199, Karnataka, India. monikasadananda@gmail.com
-
Received: ,
Accepted: ,
How to cite this article: Inavally AT, Sadananda M. Differential strain sensitivity to low-dose anxiolytic Diazepam in anxiety-related behaviour in male Wistar and Wistar-Kyoto, a rat model of endogenous depression. Indian J Physiol Pharmacol. 2024;68:108-17. doi: 10.25259/IJPP_431_2023
Abstract
Objectives:
Treatment resistant depression (TRD) prevails among individuals with major depressive disorder (MDD) with comorbid anxiety. The Wistar Kyoto (WKY) rat strain, which demonstrates exaggerated vulnerability to anxiety, has recently been suggested as a model for TRD with similar pathophysiology to MDD, non-responsiveness to antidepressants but responsive to deep brain stimulation and ketamine. At the clinical level, TRD is associated with reduced occipital cortical levels of Gamma-AminoButyric Acid (GABA), with a reduction in spontaneous GABAergic synaptic activity reported in WKY. Diazepam (DZP), a GABA agonist, is a widely used anxiolytic, so the present study was carried out to evaluate its efficacy through a low dose, oral administration in male WKY rats, with the progenitor strain Wistar, serving as vehicular control.
Materials and Methods:
Adult Wistar and WKY rats were treated with 1 mg/kg body weight DZP administered per os (p. o.) for 10 days. From the 6th day, rats were exposed to a comprehensive battery of behavioural paradigms, including novelty-based open field (OPF), anxiogenic elevated plus maze (EPM), light-dark box (LDB) and the stress coping behaviour assessing forced swim test (FST).
Results:
DZP reversed the EPM-induced anxiety in Wistars by increasing open-arm duration (P < 0.05), entries (P < 0.05) and exploratory behaviour (P < 0.01) while concomitantly decreasing closed-arm duration (P < 0.05) and entries (P < 0.05), with no effect in WKY. DZP also reduced latency to the dark zone (P < 0.05) in LDB and increased swimming behaviour in FST (P < 0.05) in Wistars, with no effect in WKY. Baseline strain differences were observed with reduced exploratory behaviour in OPF (P < 0.01), open arm entries (P < 0.01) and head dips (P < 0.01) in EPM and swimming (P < 0.05) in FST in WKY as compared to Wistars. Strain differences persisted also in the DZP-treated groups where, as compared to matched Wistars, WKY demonstrated reduced open arm duration (P < 0.05), entries (P < 0.001) and head dips (P < 0.001) and increased closed arm duration (P < 0.001) and entries (P < 0.001) in the EPM. WKY also showed reduced time spent (P < 0.05) and entries (P < 0.01) into the light zone and increased time spent in the dark zone (P < 0.05) of LDB. Further, WKY showed increased immobility (P < 0.05) during habituation and reduced swimming behaviour (P < 0.001) during the test.
Conclusion:
Strain-specific differences and increased baseline anxiety levels in WKY, as compared to Wistars, induced differential effects of DZP with drug-induced effects observed in Wistars but not in WKY, furthering the treatment resistant aspect of this model. DZP efficacy, therefore, varies in different rat strains and manifests in differential strain-specific responses emanating from exaggerated vulnerability to stress. Results also indicated differential sensitivity of tested paradigms to the anxiolytic activity of DZP and stressed the use of a battery of tests that enable a teasing out of anxiety and depression. However, further studies are needed that would unravel GABAergic differences at the receptor level, such as differential receptor binding affinities, underlying gene polymorphisms and the implications thereof for this TRD model.
Keywords
Wistar Kyoto
Treatment resistant depression
Depression
Anxiety
Diazepam
INTRODUCTION
Treatment resistant depression (TRD) exists in major depressive disorder (MDD) with over 66% failing to respond to a minimum of two prior treatments of selective serotonin reuptake inhibitors or tricyclic antidepressants posing a harder-to-treat depression with resistance manifesting with treatment ineffectiveness.[1] At the clinical level, the occurrence of anxiety leads to the exacerbation of suicidal events.[2] In such a scenario, preclinical studies are imperative for understanding drug efficacy using endogenous and induced models.
Ethologically relevant rodent paradigms are tools to investigate anxiety- and depression-like behaviours and define the preclinical efficacy of drugs.[3] Unconditioned conflict tests such as elevated plus maze (EPM), light-dark box (LDB), and open field (OPF) rely on exploratory behaviour to assess anxiety.[3] Thus, putative animal models can be classified based on drug sensitivity or resistance. Previous studies have reported anxiolytic actions of diazepam (DZP), a benzodiazepine in Wistar and other strains.[4-6] However, inbred strains such as Wistar Kyoto (WKY), which exhibit higher levels of anxiety, could provide newer insights into differential sensitivity and efficacy.[7-9]
WKY exhibits pronounced anxiety- and depressive-like behaviours, showing hypoactivity in the OPF, anxiety in the EPM and learned helplessness in the forced swim test (FST) as compared to the parent Wistar strain.[10-12] WKY has recently been suggested as a model of TRD with similar pathophysiology as in the case of MDD[13] demonstrating resistance to fluoxetine,[14,15] partial resistance to desipramine and monoamine oxidase inhibitors,[14] thus meeting major criteria of TRD such as non-responsiveness to antidepressants but responsiveness to treatment such as deep brain stimulation and ketamine.[16] Genetic variabilities reported in WKY also influence reactivity to drugs.[14,17] Reduction in spontaneous GABAergic synaptic activity was observed in WKY as compared to Wistars.[18] Since reduced GABA levels were observed in TRD patients,[19] we aimed to ascertain if the GABA agonist DZP has any effect on the WKY strain.
Dose response effects on strain have been previously studied.[4-6] DZP produced anxiolytic effects at 1.0–1.5 mg/kg,[6] while at 2 mg/kg, it induced sedation.[20] However, strain-specific differences have not been demonstrated between Wistar and WKY. Although females have a higher incidence of TRD, due to the hormonal and physiological changes that affect the action of drugs,[21] only males were used here. Therefore, strain-specific differences to 1 mg/kg DZP were assessed in male WKY as compared to Wistars to investigate its modification of symptoms in WKY strain, as they demonstrate comorbidity and meet minor criteria of TRD.
MATERIALS AND METHODS
Animals
Adult male Wistar and WKY rats (90-days-old, weighing 200.49 ± 5.59 g) were procured from Indian Council of Medical Research (ICMR)- National Institute of Nutrition (NIN)’s, Division of Animal Facility, Hyderabad, India. Animals were group-housed under standard housing conditions (24 ± 2°C; light/dark cycle of 12:12 h) with food and water supply ad libitum, and experiments conducted as per Committee for the Purpose of Control and Supervision of Experiments on Animals (CPCSEA) guidelines after due approval from the Institutional Animal Ethics Committee (IAEC) (MU/CARRT/IAECMU-RP-3/4 dt. 9 August 2021). After 1 week of acclimatisation and 3 days of handling, 1 mg/kg b.w. DZP dissolved in distilled water (Lori®, Neon Labs Ltd., Mumbai) was administered by oral gavage for 5 consecutive days and continued to be administered 30 min before each test till the 10th day as half-life in rodents is 1.5 h, and serum DZP levels have been shown to increase and plateau after approximately the 7th day of continuous dosing in humans.[22,23] Controls were intubated with the vehicle.
Behavioural analysis
From the 6th day, rats were put through a battery of tests conducted at 24 h intervals: OPF, EPM, LDB and FST. The tests were ordered based on increasing stress levels [Figure 1]. Behaviours were recorded using a Panasonic WV CP500 camera fed to a Piccolo frame grabber card and analysed automatically using Ethovision XT version 8.0® (Noldus, Netherlands).
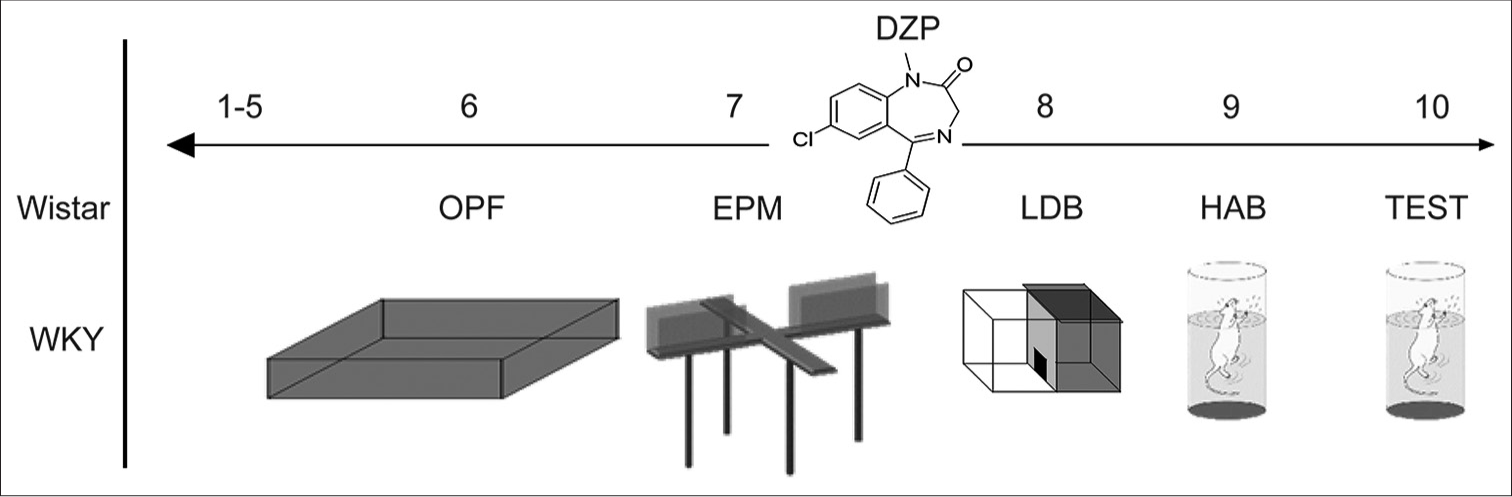
- Experimental design: diazepam was administered to both Wistars and Wistar Kyoto (WKY). Animals were handled from day 1 to 5 and tested on the open field, elevated plus maze, light dark box and forced swim test on consecutive days thereafter. DZP: Diazepam, OPF: open field; EPM: elevated plus maze; LDB: light dark box; HAB: habituation phase of forced swim test; TEST: test phase of forced swim test.
OPF
Animals were placed individually in the centre of the OPF, which consisted of a square apparatus made of plexiglass with dimensions 100 × 100 cm, 40 cm high. The apparatus was wiped with 1% acetic acid after each trial. Duration, number of entries to the centre and periphery and distance travelled were recorded automatically. Grooming, rearing and faecal boli were scored manually.
EPM
The EPM was raised 50 cm above the floor and consisted of two open (50 × 10 cm) and two closed roofless arms (50 × 10 × 40 cm) perpendicular to each other linked by central zone (10 × 10 cm). Animals were placed individually in the centre facing the open arm and allowed to explore for 5 min. Measures taken were distance moved, open and closed arm duration, entries and latency,[11,12] which were recorded automatically. Other ethological parameters such as head dips (where the rat protrudes its head and scans the floor from the sides of the open arm), rearing, stretch-attend postures (where the rat stretches forward to the open arm, maintains a flat-back posture and retracts back to its original position) and grooming were scored manually.[6] Anxiety index[24] was calculated using the formula 1 − (open arm entry/total entry + open arm duration/total duration/2).
LDB
The LDB apparatus consisted of the brightly illuminated chamber (350 lux; 25 × 25 × 50 cm) and a similar sized dark chamber (8–10 lux; 25 × 25 × 50 cm) connected by a small doorway for the rats to move freely between the compartments. Animals were placed individually in the light chamber and allowed to explore freely for 5 min. Time spent in each compartment, number of entries and latency were recorded.
FST
FST included two sessions: habituation and test. The rats were placed individually in a cylindrical water tank (40 cm diameter, 44 cm height) and allowed to swim for 15 min (habituation). 24 h later, rats were tested for 5 min.[12] Immobility (floating without struggling), latency to immobility, swimming, and climbing behaviours were scored.
Statistical analysis
Data are expressed as Mean ± standard error of the mean. Two-way analysis of variance (ANOVA) for strain and drug effects with Bonferroni’s post-test was applied. P-value was set at <0.05. Pearson’s correlation analysis was used to assess measures across tests. Statistical analysis and graphs were performed using Graph Pad Prism 5.01.
RESULTS
Two-way ANOVA analysis revealed some main effects of the treatment and strain. Significant interactions between treatment and strain were also observed.
OPF
Main effects of treatment, strain or interaction were not observed on time spent or entries into the centre and periphery [Figure 2a-d]. However, main effect of strain (F(1,28) = 16.31; P = 0.0004) was observed on exploratory behaviour with significantly reduced rearings in vehicular WKY as compared to matched Wistars (P < 0.01; [Figure 2e]). A significant main effect of strain was also observed on defecation (F (1,28) = 5.49; P = 0.02) with a significant increase in defecation in DZP-treated WKY as compared to matched Wistars (P < 0.05; [Figure 2f]).
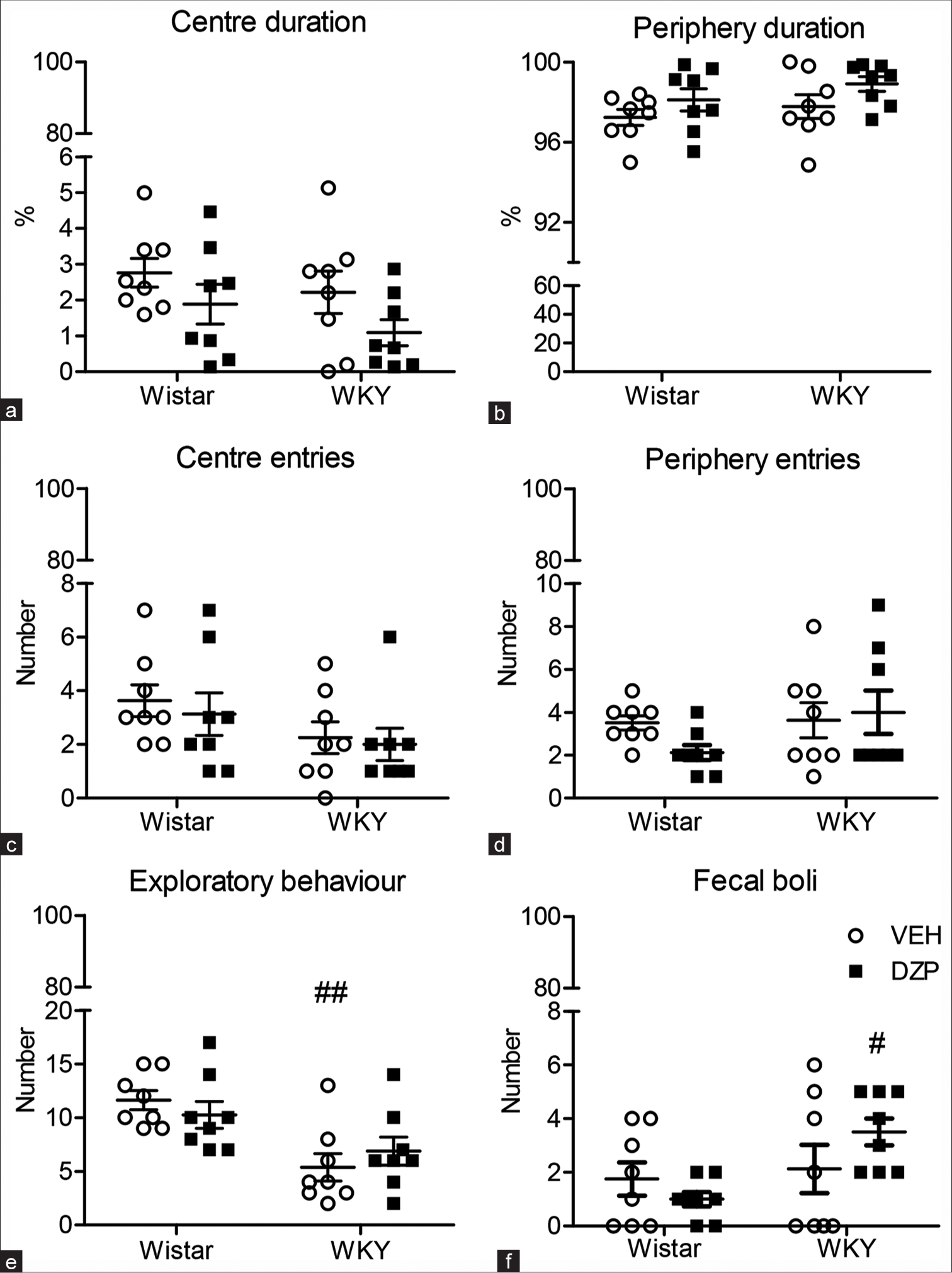
- Effect of diazepam on anxiety-like behaviours in the OPF in Wistar and Wistar Kyoto strains (WKY). The open circles represent the vehicular controls while squares represent the diazepam treated groups. Parameters depicted are (a) centre and (b) periphery duration; (c) centre and (d) periphery entries; (e) exploratory behavior; (f) fecal boli in the OPF for 5 min. Controls were intubated with vehicle. Data are expressed as mean ± standard error of the mean. Two-way analysis of variance followed by post-test: #represents strain differences; #P <0.05; ##P < 0.01. (a-f) ‘o’ indicates VEH and ‘■’ indicates DZP. VEH: vehicle, DZP: Diazepam.
EPM
DZP had a significant effect (F (1,28) = 6.66; P = 0.01) on open arm time with an increase in open arm time in DZP-treated Wistars as compared to vehicular controls (P < 0.05). Significant effects of strain (F (1,28) = 11.50; P = 0.002) were also observed with DZP-treated WKY spending significantly reduced time in the open arms (P < 0.05) as compared to matched Wistars [Figure 3a]. DZP effect (F (1,28) = 7.46; P = 0.01) was also observed in closed arm duration [Figure 3b] with significant reduction in closed arm time (P < 0.05) in DZP-treated Wistars as compared to vehicular controls. Significant effects of strain (F (1,28) = 21.17; P < 0.0001) were also observed in closed arm duration [Figure 3b] with significantly increased closed arm time (P < 0.001) in DZP-treated WKY as compared to matched Wistars.
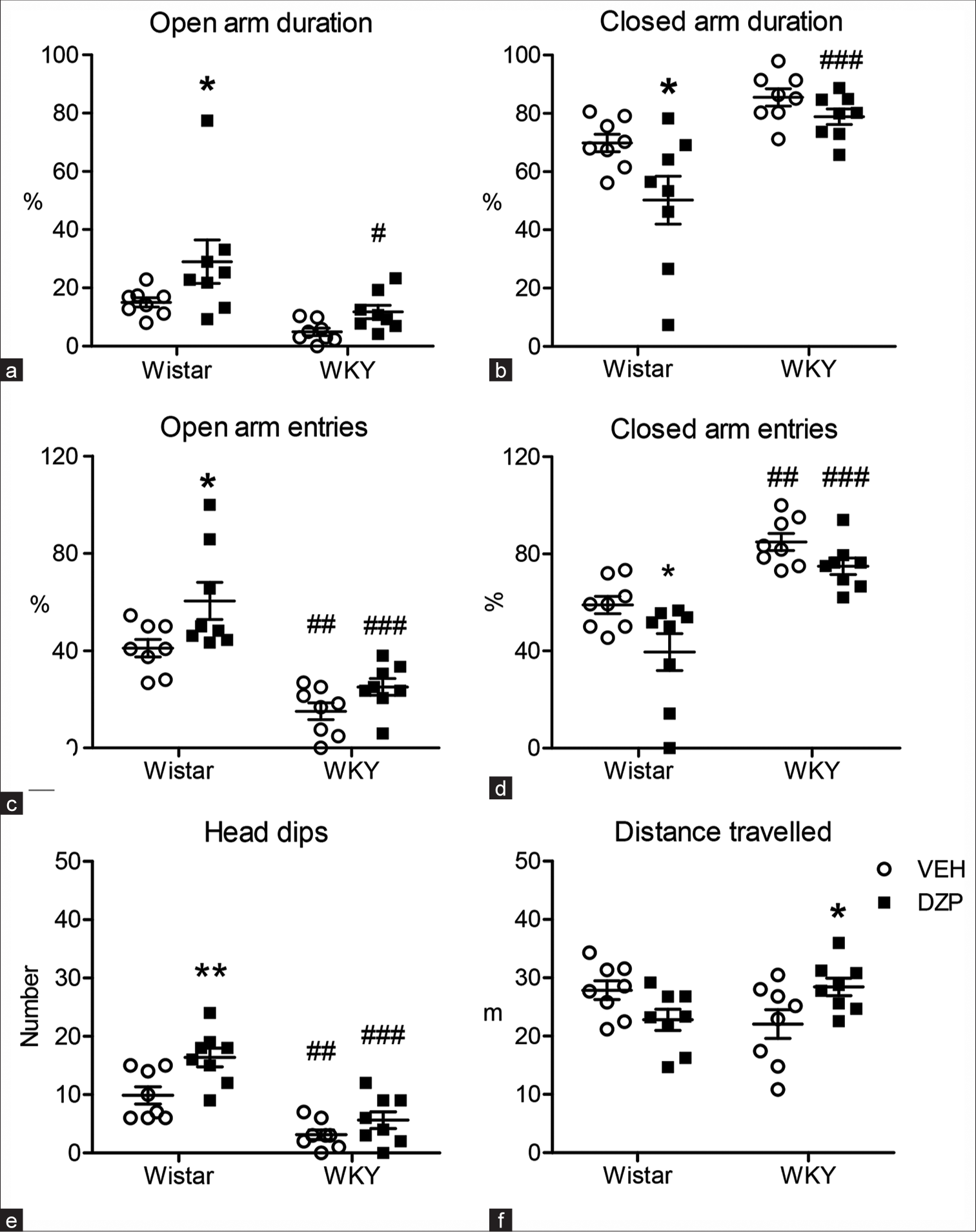
- Effect of diazepam on anxiety neurobehaviours in the elevated plus maze (EPM) in Wistar and Wistar Kyoto strains (WKY). The open circles represent the vehicular controls while squares represent the diazepam treated groups. Parameters depicted are (a) open arm and (b) closed arm duration; (c) open arm and (d) closed arm entries; (e) head dips and (f) distance travelled in the EPM for 5 min. Controls were intubated with vehicle. Data are expressed as mean ± standard error of the mean. Two-way analysis of variance followed by post-test: * represents effects of the drug; # represents strain differences; * and # P < 0.05; ** and ## P < 0.01; ### P < 0.001. (a-f) ‘o’ indicates VEH and ‘■’ indicates DZP. VEH: vehicle, DZP: Diazepam
DZP effect (F (1,28) = 9.12; P = 0.005) was also observed in the frequency of entries into both arms with significantly reduced closed and concomitantly increased open arm (P < 0.05) entries in DZP-treated Wistars [Figure 3c and d] as compared to controls. A significant effect of strain (F (1,28) = 39.77; P < 0.0001) was observed on a number of entries into both arms, with WKY demonstrating reduced open arm entries (P < 0.01), also on DZP treatment (P < 0.001) [Figure 3c] and concomitantly increased closed arm entries [Figure 3d] as compared to matched Wistars.
DZP effect (F (1,28) = 10.66; P = 0.002) was observed on head dips [Figure 3e] with a significant increase in DZP-treated Wistars (P < 0.01) as compared to vehicular controls. Significant effects of strain (F (1,28) = 40.31; P < 0.0001) were observed on head dips [Figure 3e] with vehicular (P < 0.01) and DZP-treated WKY (P < 0.001) demonstrating reduced head dips [Figure 3e] as compared to matched Wistars. Distance travelled is shown in Figure 3f.
Overall, anxiety levels calculated as anxiety index [Table 1] were significantly affected by DZP treatment (F (1,28) = 12.40; P = 0.001) with DZP inducing a significant reduction in anxiety levels in Wistars (P < 0.01). Anxiety levels were also affected by strain (F (1,28) = 28.28; P < 0.0001) with vehicular (P < 0.05) and DZP-treated (P < 0.001) WKY demonstrating increased anxiety [Table 1] as compared to matched Wistars.
Wistar | WKY | ||||
---|---|---|---|---|---|
VEH | DZP | VEH | DZP | ||
Anxiety index | 0.22±0.02 | −0.04±0.09** | 0.39±0.02# | 0.31±0.02### | |
Rearing (no.) | 10.00±1.05 | 9.00±0.73 | 8.50±1.28 | 11.75±1.58 | |
SAP (no.) | 3.88±0.81 | 4.88±1.77 | 4.88±0.72 | 4.50±0.65 | |
Grooming (s) | 37.13±5.22 | 26.88±11.23 | 40.63±12.45 | 28.88±6.98 | |
Fecal boli (no) | 0.50±0.19 | 1.50±0.68 | 2.75±1.08 | 2.25±0.49 |
Data are presented as Mean±SEM. #: Indicates strain-specific differences. #P< 0.05; **P< 0.01; ###P< 0.001. SAP: Stretch-attend postures. For definition of anxiety index and SAP, see materials and methods. WKY: Wistar Kyoto, VEH: vehicle, DZP: Diazepam
For all other parameters such as stretch-attend postures, rearing frequency, time spent grooming and defecation in the EPM, see Table 1.
LDB
Significant effects of the strain (F (1,28) = 4.74; P = 0.038) were observed with DZP-treated WKY spending significantly less time in the light zone and concomitantly more time in the dark zone (P < 0.05; [Figure 4a and b] as also reduced entries into light zone (P < 0.01;) (F (1,28) = 14.14; P = 0.0008 [Figure 4c]) as compared to matched Wistars. DZP had a significant effect (F (1,28) = 5.99; P = 0.02) on latency to enter the dark zone, which was significantly reduced in DZP-treated Wistars as compared to controls (P < 0.05; [Figure 4d]).
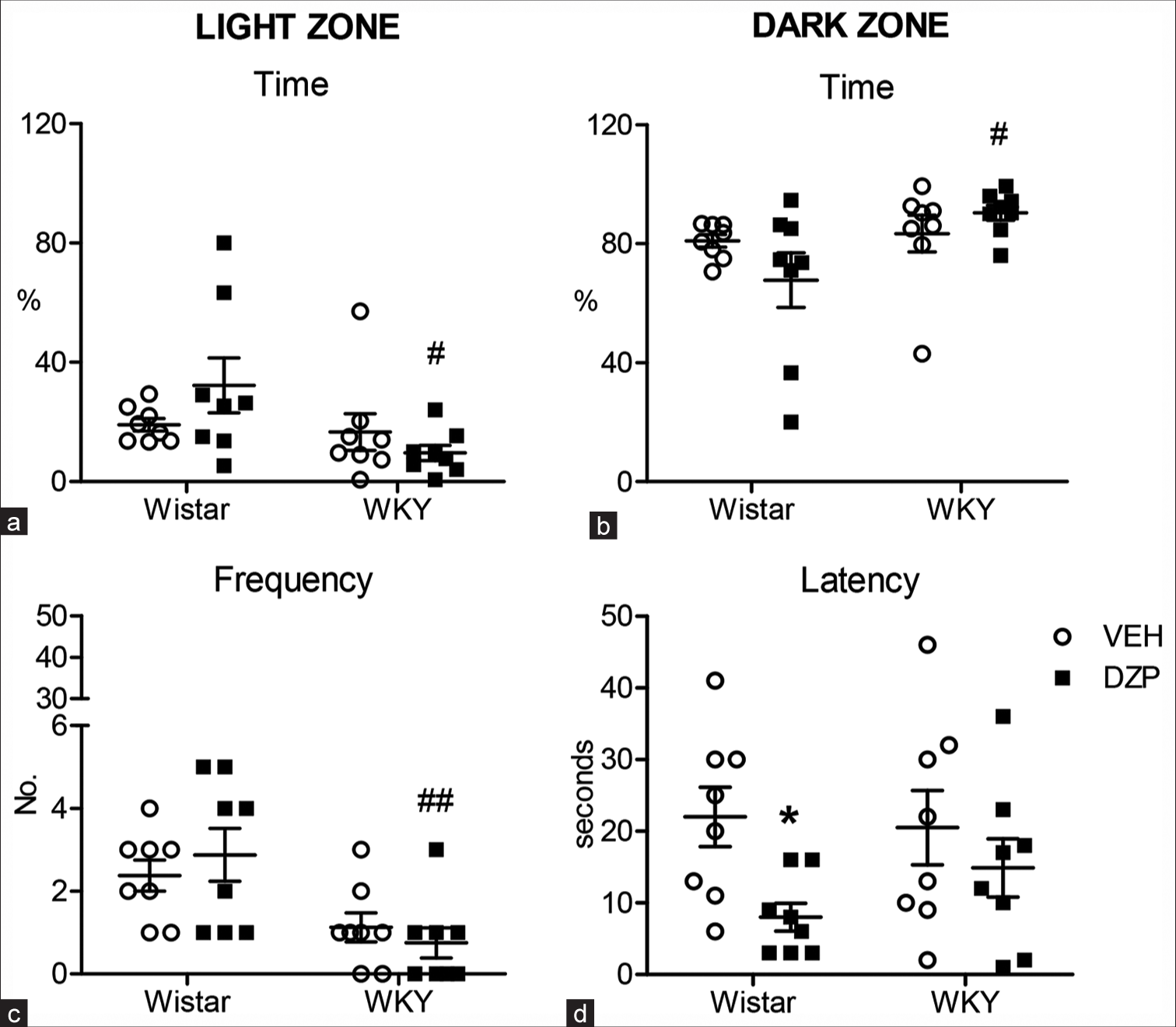
- Effect of diazepam on neurobehaviours in light-dark box in Wistar and Wistar Kyoto (WKY) strains. The open circles represent the vehicular controls while squares represent the treated groups. Parameters depicted are time spent in the (a) light and (b) dark zones; (c) frequency of entries into light zone; (d) latency to enter dark zone. Data are expressed as mean ± standard error of the mean. Two-way analysis of variance followed by post-test: *represents significant drug effects; # indicates significant strain-specific differences; *, # P < 0.05; ## P < 0.01. (a-d) ‘o’ indicates VEH and ‘■’ indicates DZP. VEH: Vehicle, DZP: Diazepam.
FST – Habituation
Strain differences (F (1,28) = 7.50; P = 0.01) were observed in DZP-treated groups with a significant decrease in immobility in DZP-treated Wistars as compared to matched WKY (P < 0.05; [Figure 5a]). WKY demonstrated significantly reduced swimming behaviour as compared to matched Wistars (P < 0.05; F (1,28) = 12.47; P = 0.001; [Figure 5b]). DZP-treated WKY demonstrated significantly (P < 0.01) reduced climbing behaviour [Figure 5c] as compared to vehicular controls, revealing a significant effect of the treatment (F (1,28) = 4.31; P = 0.04) with significant strain x treatment interaction (F (1,28) = 5.09; P = 0.03).
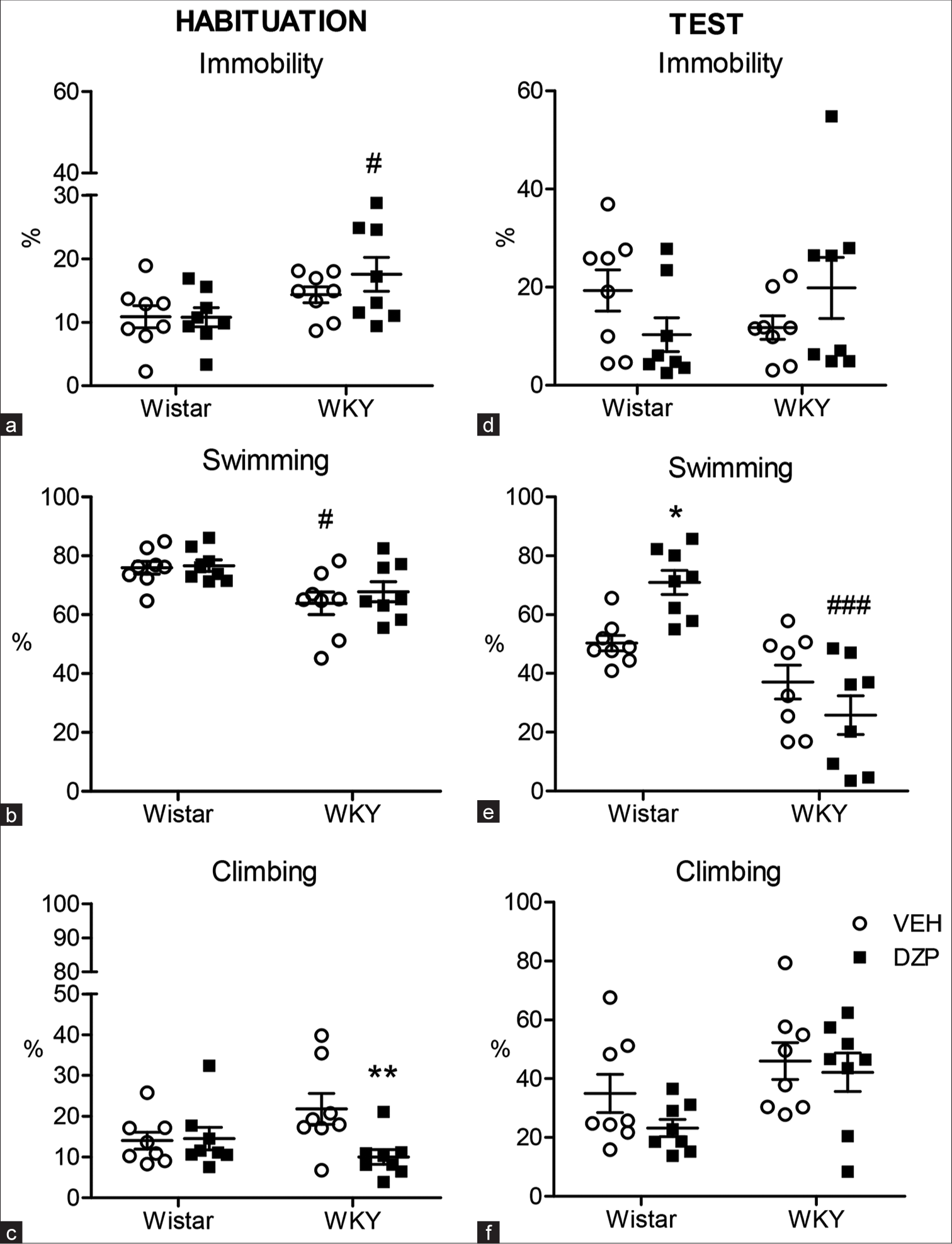
- Effect of diazepam on depressive-like behaviours in the forced swim test. The open circles represent the vehicular controls while squares represent the diazepam treated groups. Left panel depicts measures during habituation. Right panel depicts same measures recorded during the test. For more details see Methods. Left panel (a,b,c) depict immobility, swimming, and climbing duration during habituation. Right panel (d,e,f) depict the same measures recorded during test. Data are expressed as Mean ± standard error of the mean. *Represents the significant drug effects; # indicates significant strain-specific differences; *, # P < 0.05; ** P < 0.01; ### P < 0.001. (a-f) ‘o’ indicates VEH and ‘■’ indicates DZP. VEH: Vehicle, DZP: Diazepam.
FST – TEST
No effects of strain, treatment or interaction were observed on immobility duration during the test [Figure 5d]. DZP-treated Wistars demonstrated significantly increased swimming behaviour as compared to vehicular controls (P < 0.05) with significant strain x treatment interaction (F (1,28) = 10.15; P = 0.003). The main effect of strain was observed on swimming behaviour with a significant decrease in swimming behaviour in treated WKY as compared to matched Wistars (P < 0.001; F (1,28) = 33.96; P < 0.0001; [Figure 5e]). Although significant strain effects (F (1,28) = 6.76; P = 0.01) were observed on climbing behaviour, no individual group differences were seen [Figure 5f].
Correlation
Total entries into centre and periphery areas in OPF and into open and closed arms of EPM were positively correlated across all groups: Wistars (r = 0.78; P = 0.04), DZP-treated Wistars (r = 0.81; P = 0.03), WKY (r = 0.73; P = 0.04) and DZP-treated WKY (r = 0.84; P = 0.019). In vehicular Wistars, centre time and periphery time in OPF were positively correlated with open arm (r = 0.80; P = 0.01) and closed arm duration (r = 0.76; P = 0.01) in EPM, respectively.
DISCUSSION
Results indicate that DZP efficacy depends on the strain used, as anxiolytic effects were observed in Wistars in the EPM and, to some extent, in LDB and FST, with no or very little effect on OPF. However, no effect was observed in the WKY strain, indicating the influence of baseline anxiety.[4,5] As WKY demonstrates exaggerated anxiety-like behaviour[8] and meets minor criteria of TRD,[13] the results obtained here enable an understanding of DZP in TRD with comorbid anxiety. Previous dose-dependent studies have shown that while DZP at 0.8 mg/kg had no anxiolytic effect on overt behaviour in the OPF in male Wistars,[25] a reduction in anxiety levels was observed at 1 mg/kg with no effect on locomotion,[26] while 1.5 or 1 mg/kg had similar anxiolytic effects in EPM[6] and 2 mg/kg was reported to produce sedative and amnesic effects.[20]
No effect of DZP was observed in OPF in both Wistar and WKY, which could have been due to the size of the OPF, as WKY has been shown to be hypoactive as compared to Wistars.[10] Rearing, a measure of anxiety[27] was decreased in WKY, while defecation was increased in DZP-treated WKY as compared to matched Wistars, indicating anxiety-like profile.
In the anxiogenic EPM, DZP’s anxiolytic effects were observed in Wistars, while distance travelled was similar, indicating that locomotory behaviour was not affected and that the effects observed in Wistars and non-responsiveness in WKY did not result from DZP’s sedative property. The anxiolytic effect in Wistars is in line with other studies where the same dose was used ip., enabling a distinction between low- and high-anxiety rats.[4] As expected, increased anxiety scores were observed in WKY, which could have reduced the sensitivity of EPM to DZP[28] as 1mg/kg induces anxiolytic effects in other strains.
In LDB, which is mainly used to assess the anxiolytic effect,[29] DZP had no effect in either Wistars or WKY. However, latency to enter the dark zone was reduced on DZP treatment in Wistars, indicating hyperactivity, while increased anxiety-like behaviour was observed in WKY. Strain specific-differences in drug sensitivity may be due to strain-specific phenotypic characteristics, variation in neurobiological constructs and interaction of the model with extraneous factors. This is evident particularly in the EPM that measures innate or trait anxiety compounded by state/context and is based on the conflict between the animal’s innate tendency to approach open spaces vis-a-vis its innate urge to remain in sheltered spaces of the closed arms.[6,30,31]
DZP had no effect on time spent immobile in the FST in both the strains which are in line with the previous studies using acute administration,[4,32] suggesting that these behaviours reflect state, rather than anxiety or fear. However, DZP increased swimming behaviour in Wistars, but not in WKY, indicating differential drug sensitivity. Additional effects of strain were also observed on climbing behaviour, which could have been contributed by increased re-emergence activity from diving/submergence in WKY, indicating adaptive or coping behaviour.[33]
Each paradigm elicits different behaviours and increases the reliability of the results, with heterogeneity, procedural variables and laboratory factors affecting comparison across paradigms.[31] Overall, the sensitivity of DZP may differ based on the paradigm’s variability in assessing different aspects of anxiety-like neurobehaviours.[34]
DZP was effective in Wistars, the control strain, but was ineffective in WKY, a model of endogenous depression. This is indicative of differential sensitivities to the drug, as also observed in drug transport capacity, bioavailability, phasic response, bimodal effects, etc., making certain strains more or less suitable for evaluating the pharmacokinetics of drugs.[35] Heterogeneity in WKY expressing variability to stress has been reported [36] with selectively bred WKY highly immobile rats displaying hyperresponsiveness, while WKY least immobile rats show only partial responsiveness in FST on treatment with antidepressants and during the assessment of resistance to fluoxetine.[14] In fact, genomic screening showed differential genes associated with stress and antidepressant mechanisms, highlighting strain-specific differential sensitivity to drugs.[37]
The ineffectiveness of DZP to WKY could be due to strain differences in underlying GABAA receptor sensitivity, as WKY demonstrate reduced spontaneous GABAergic synaptic activity associated with dysregulation of inhibitory control by GABAergic input on neurons of the locus coeruleus as compared to Wistars.[18] Furthermore, strain differences have been observed in GABAA receptor binding with higher binding in amygdalar, hippocampal (CA2, CA3, dentate gyrus) and caudate putamen brain areas of WKY as compared to Wistars.[38] The order of behavioural paradigms used could not have affected the results, as comparable locomotion and exploratory activity[39] were observed across groups in both OPF and EPM.
As the battery of tests employed enables measurement of various facets of anxiety and depression, teases out anxiety from depression-related symptoms and elicits varied drug sensitivities, personalised approaches in the treatment of anxiety and depression by assessing its severity, nature of symptoms, the system’s interaction with the drug and individual variations among patients of MDD comorbid with anxiety are essential.[40] The major limitation encountered during interpretation was the single dose used. However, as other studies had demonstrated sedation effects already at 2 mg/kg[20] and as 1–1.5 mg/kg dosage had shown similar effects,[6] a single low-dose design was considered to assess differential strain-specific responses to DZP. However, only a more comprehensive gene expression study of differential GABA receptors and sensitivities or thresholds would substantiate our results.
CONCLUSION
Our results indicated strain-specific differences to DZP treatment and corroborated evidence for WKY as a model for TRD resulting from underlying increased baseline anxiety levels. Differential effects of DZP with drug-induced effects observed in Wistars, but not in WKY, point to variable efficacy of DZP that manifests in differential strain-specific responses emanating from exaggerated vulnerability to stress, as in the case of WKY. Sensitivity to DZP also varied in different paradigms tested, validating the use of a battery of tests to measure anxiety- and depression-related symptoms. Further studies, however, are necessary to unravel receptor level differences, binding affinities and underlying gene polymorphisms for therapeutic implications in TRD.
Acknowledgement
The first author is thankful to the Department of Biotechnology (DBT), Government of India, New Delhi for providing DBT-JRF fellowship (No. DBTHRDPMU/JRF/BET-20/I/2020/AL/361 dated 05 February 2021).
Ethical approval
The study is approved from the Institutional Animal Ethics Committee (IAEC) (MU/CARRT/IAECMU-RP-3/4 dated 9 August 2021).
Declaration of patient consent
Patient’s consent was not required as there are no patients in this study.
Conflicts of interest
There are no conflicts of interest.
Use of artificial intelligence (AI)-assisted technology for manuscript preparation
The authors confirm that there was no use of artificial intelligence (AI)-assisted technology for assisting in the writing or editing of the manuscript and no images were manipulated using AI.
Financial support and sponsorship
Nil.
References
- Defining treatment-resistant depression. Depress Anxiety. 2020;37:134-45.
- [CrossRef] [PubMed] [Google Scholar]
- Investigation of early and lifetime clinical features and comorbidities for the risk of developing treatment-resistant depression in a 13-year nationwide cohort study. BMC Psychiatry. 2020;20:541.
- [CrossRef] [PubMed] [Google Scholar]
- Experimental animal models for the simulation of depression and anxiety. Dialogues Clin Neurosci. 2022;8:323-33.
- [CrossRef] [PubMed] [Google Scholar]
- Behavioral, physiological, and neuroendocrine stress responses and differential sensitivity to diazepam in two Wistar rat lines selectively bred for high-and low-anxiety-related behavior. Neuropsychopharmacology. 1998;19:381-96.
- [CrossRef] [PubMed] [Google Scholar]
- Different effects of diazepam in Fischer rats and two stocks of Wistar rats in tests of anxiety. Pharmacol Biochem Behav. 2001;70:411-20.
- [CrossRef] [PubMed] [Google Scholar]
- A comparison between Dark Agouti and Sprague-Dawley rats in their behaviour on the elevated plus-maze, open-field apparatus and activity meters, and their response to diazepam. Psychopharmacology (Berl). 2002;159:188-95.
- [CrossRef] [PubMed] [Google Scholar]
- Measuring normal and pathological anxiety-like behaviour in mice: A review. Behav Brain Res. 2001;125:141-9.
- [CrossRef] [PubMed] [Google Scholar]
- Wistar-Kyoto rats as an animal model of anxiety vulnerability: Support for a hypervigilance hypothesis. Behav Brain Res. 2009;204:162-8.
- [CrossRef] [PubMed] [Google Scholar]
- Evaluation of the Wistar-Kyoto rat model of depression and the role of synaptic plasticity in depression and antidepressant response. Neurosci Biobehav Rev. 2019;105:1-23.
- [CrossRef] [PubMed] [Google Scholar]
- Open field, learned helplessness, conditioned defensive burying, and forced-swim tests in WKY rats. Physiol Behav. 1994;55:433-9.
- [CrossRef] [PubMed] [Google Scholar]
- Anxiety-and depressive-like profiles during early-and mid-adolescence in the female Wistar Kyoto rat. Int J Dev Neurosci. 2017;56:18-26.
- [CrossRef] [PubMed] [Google Scholar]
- Estrous cycle phase-dependent changes in anxiety-and depression-like profiles in the late adolescent Wistar-Kyoto rat. Ann Neurosci. 2017;24:136-45.
- [CrossRef] [PubMed] [Google Scholar]
- The Wistar-Kyoto rat model of endogenous depression: A tool for exploring treatment resistance with an urgent need to focus on sex differences. Prog Neuropsychopharmacol Biol Psychiatry. 2020;101:109908.
- [CrossRef] [PubMed] [Google Scholar]
- Selectively bred Wistar-Kyoto rats: An animal model of depression and hyper-responsiveness to antidepressants. Mol Psychiatr. 2003;8:925-32.
- [CrossRef] [PubMed] [Google Scholar]
- The effects of perinatal fluoxetine exposure on emotionality behaviours and cortical and hippocampal glutamatergic receptors in female Sprague-Dawley and Wistar-Kyoto rats. Prog Neuropsychopharmacol Biol Psychiatry. 2021;108:110174.
- [CrossRef] [PubMed] [Google Scholar]
- Validation of chronic mild stress in the Wistar-Kyoto rat as an animal model of treatment-resistant depression. Behav Pharmacol. 2019;30:239-50.
- [CrossRef] [PubMed] [Google Scholar]
- Strain differences in the behavioral effects of antidepressant drugs in the rat forced swimming test. Neuropsychopharmacology. 2000;22:191-9.
- [CrossRef] [PubMed] [Google Scholar]
- Dysfunctional inhibitory mechanisms in locus coeruleus neurons of the Wistar Kyoto Rat. Int J Neuropsychopharmacol. 2015;18:pyu122.
- [CrossRef] [PubMed] [Google Scholar]
- Amino acid neurotransmitters assessed by proton magnetic resonance spectroscopy: Relationship to treatment resistance in major depressive disorder. Biol Psychiatry. 2009;65:792-800.
- [CrossRef] [PubMed] [Google Scholar]
- Behavioral analysis of diazepam-induced memory deficits: Evidence for sedation-like effects. Psychopharmacology. 1992;106:297-302.
- [CrossRef] [PubMed] [Google Scholar]
- Effect of estrous cycle on behavior of females in rodent tests of anxiety. Front Psychiatry. 2021;12:711065.
- [CrossRef] [PubMed] [Google Scholar]
- The pharmacokinetics of diazepam and desmethyldiazepam in rat brain and plasma. Psychopharmacology. 1986;88:267-70.
- [CrossRef] [PubMed] [Google Scholar]
- Pharmacokinetic profile of diazepam in man following single intravenous and oral and chronic oral administrations. J Pharm Sci. 1973;62:1789-96.
- [CrossRef] [Google Scholar]
- Early post-stressor intervention with high-dose corticosterone attenuates posttraumatic stress response in an animal model of posttraumatic stress disorder. Biol Psychiatry. 2008;64:708-17.
- [CrossRef] [PubMed] [Google Scholar]
- Effects of buspirone, diazepam, and zolpidem on open field behavior, and brain [3H] muscimol binding after buspirone pretreatment. Pharmacol Biochem Behav. 2000;66:645-51.
- [CrossRef] [PubMed] [Google Scholar]
- Novel and simple behavioral paradigm for assessing anxiety in rats: Effect of diazepam. Neuroendocrinol Lett. 2009;30:25-31.
- [Google Scholar]
- Exploratory rearing: A context-and stress-sensitive behavior recorded in the open-field test. Stress. 2018;21:443-52.
- [CrossRef] [PubMed] [Google Scholar]
- Variations in illumination, closed wall transparency and/or extramaze space influence both baseline anxiety and response to diazepam in the rat elevated plus-maze. Behav Brain Res. 2009;203:35-42.
- [CrossRef] [PubMed] [Google Scholar]
- The mouse light-dark box test In: Gould TD, ed. Mood and anxiety related phenotypes in mice. Totowa, NJ: Humana Press; 2009. p. :197-223.
- [CrossRef] [Google Scholar]
- Trait and state anxiety in animal models: Is there correlation? Neurosci Lett. 2009;450:266-9.
- [CrossRef] [PubMed] [Google Scholar]
- What are we measuring when we test strain differences in anxiety in mice? Behav Genet. 2013;43:34-50.
- [CrossRef] [PubMed] [Google Scholar]
- Pharmacological validation of a chronic social stress model of depression in rats: Effects of reboxetine, haloperidol and diazepam. Behav Pharmacol. 2008;19:183-96.
- [CrossRef] [PubMed] [Google Scholar]
- Immobility in the forced swim test is adaptive and does not reflect depression. Psychoneuroendocrinology. 2015;62:389-91.
- [CrossRef] [PubMed] [Google Scholar]
- Differences in anxiety-related behaviours and in sensitivity to diazepam in inbred and outbred strains of mice. Psychopharmacology. 2000;148:164-70.
- [CrossRef] [PubMed] [Google Scholar]
- Strain differences in the drug transport capacity of intestinal glucose transporters in Sprague-Dawley versus Wistar rats, C57BL/6J versus Kunming mice. Int J Pharmaceut. 2023;640:123000.
- [CrossRef] [PubMed] [Google Scholar]
- Chronic unpredictable mild stress for modeling depression in rodents: Meta-analysis of model reliability. Neurosci Biobehav Rev. 2019;99:101-16.
- [CrossRef] [PubMed] [Google Scholar]
- Insufficiency of ventral hippocampus to medial prefrontal cortex transmission explains antidepressant non-response. J Psychopharmacol. 2021;35:1253-64.
- [CrossRef] [PubMed] [Google Scholar]
- Strain differences in the distribution of N-methyl-d-aspartate and gamma (g)-aminobutyric acid-A receptors in rat brain. Life Sci. 2009;85:794-9.
- [CrossRef] [PubMed] [Google Scholar]
- Determination of motor activity and anxiety-related behaviour in rodents: Methodological aspects and role of nitric oxide. Interdiscip Toxicol. 2013;6:126-35.
- [CrossRef] [PubMed] [Google Scholar]
- Effectiveness of a tailored implementation programme to improve recognition, diagnosis and treatment of anxiety and depression in general practice: A cluster randomised controlled trial. Implement Sci. 2015;10:33.
- [CrossRef] [PubMed] [Google Scholar]