Translate this page into:
Role of insulin in oleic acid-induced acute lung injury in rat model
*Corresponding author: Ratna Pandey, Department of Physiology, Institute of Medical Sciences, Banaras Hindu University, Varanasi, Uttar Pradesh, India. ratna93@yahoo.co.in
-
Received: ,
Accepted: ,
How to cite this article: Kumar S, Bhagat P, Patne SC, Pandey R. Role of insulin in oleic acid-induced acute lung injury in rat model. Indian J Physiol Pharmacol 2021;65(2):103-8.
Abstract
Objectives:
Acute lung injury (ALI) is an inflammatory condition, therefore, this study was undertaken to determine the effect of insulin (an immunomodulatory agent) in oleic acid (OA)-induced ALI in rat model.
Materials and Methods:
The experiments were performed on adult male albino rats (total n = 18). The trachea, jugular vein and carotid artery of anaesthetised adult rats were cannulated to keep the respiratory tract patent, deliver saline/drugs and recording of blood pressure, respectively. Animals were divided into three groups. In Group I (control group), normal saline (75 μL) was injected and this group served as control group. In Group II (OA group), OA (75 μL) was administered to induce ALI in rats. In Group III (insulin + OA), OA (75 μL) was injected in insulin pre-treated rats. Respiratory frequency (RF), heart rate (HR) and mean arterial pressure (MAP) were recorded on computerised chart recorder; arterial blood sample was collected to determine PaO2/FiO2. Further, pulmonary water content was determined, and histological examination of the lung was done in all animals.
Results:
Injection of OA produced ALI indicated by significant increase in RF by 30 min followed by progressive decrease and ultimately death of animal. Significant increase in the pulmonary water content and decrease in PaO2/FiO2 were observed in these animals. Histological examination of lungs showed damage to the lung parenchyma. An immediate decrease in HR and MAP followed by some improvement and then progressive decrease was also observed.
Conclusions:
Insulin (an immunomodulatory agent) pre-treatment delayed initiation of OA-induced ALI as indicated by protection against OA-induced severe alteration in the RF in the initial stage and less lung injury in histological examination, although; it could not alter the overall course of the disease.
Keywords
Lung injury
Immunomodulatory agents
Pulmonary oedema
Albino rat
INTRODUCTION
Acute lung injury (ALI) is acute respiratory failure with substantial morbidity and mortality.[1] ALI is a distressing condition and is well defined by the American-European Consensus Committee in patients as the acute onset of diffuse bilateral pulmonary infiltrates by chest radiograph; a PaO2/FiO2 <300 for ALI and a pulmonary artery wedge pressure <18 or no clinical evidence of left atrial hypertension.[1] ALI is well defined in human; it is not possible to directly translate the criteria of ALI in humans to experimental animals because of many anatomical and physiological differences between them. Therefore, the American Thoracic Society (ATS) in a workshop concluded that the main features of animal model of ALI include histological evidence of lung injury, alteration of the alveolar capillary barrier, presence of an inflammatory response and evidence of physiological dysfunction.[2] To determine ALI, at least three of these four main features of ALI should be present.[2] In earlier studies, oleic acid (OA) administration induced ALI in rats which was characterised by ventilatory changes, hypoxemia (decreased PaO2/FiO2 ratio), pulmonary oedema and inflammatory cells in lungs as per ATS guidelines.[3,4] Therefore, we used OA-induced ALI model in our study.
Acute inflammation is a key feature of ALI, as evidenced by the central role of neutrophils recruitment and activation.[5] Further, there are also increased expression and release of inflammatory mediators, such as pro-inflammatory and anti-inflammatory cytokines and chemokines.[6] Disturbances in surfactant function and fluid clearance from the lungs are present, and ample evidence supports imbalance in oxidant/ antioxidant activity, coagulation/fibrinolysis and fibrosis/ repair.[1,7] The relative balance of these interacting pathways either exacerbates or ameliorates further lung injury.[7]
ALI has been reported to be one of the common causes of death in critically ill patients.[8] Insulin administrations have been recommended for critically ill patients.[8,9] An earlier report of critical illness has shown that insulin has immunomodulatory effect, and insulin treatment prevents endothelial dysfunction and neutrophil chemotaxis.[10-12] Study in a rat model of endotoxin-induced ALI has shown that tight glycaemic control reduced the severity of lung injury.[13] Inflammation is a characteristic feature of ALI, so we hypothesised that an immunomodulatory agent (like insulin) may be beneficial in ALI. Therefore, the present study was undertaken to examine the role of insulin in ALI in rat model.
MATERIALS AND METHODS
Animals, anaesthesia and recording procedure
All the animal experiments were performed after approval from the Institute Ethical Committee of Institute of Medical Sciences, Banaras Hindu University, Varanasi, India (Approval letter no. Dean/2016/CAEC/621). OA-induced ALI rat model which mimics ALI in human was used for the study.[4] Adult male albino rats of Charles-Foster strain weighing (175–225 g) were selected for the study. Animals were kept in a temperature (25 ± 0.5°C), humidity (50% RH) and light controlled room (12 h:12 h, light-dark period).[3] Commercially available rat normal pellet diet (Hindustan Lever, Mumbai, India) and water were given ad libitum.[14,15] Urethane (1.5 g/kg body weight i.p.) was used to anaesthetise the animal.[3] After anaesthetising the rats, tracheal, jugular vein and carotid artery cannulation were done to keep the respiratory tract patent, saline/ drug administration and recording of blood pressure (BP), respectively. The respiratory excursions were recorded on a chart recorder through the force-displacement transducer attached with the help of thread secured to the skin over the xiphisternum to determine respiratory frequency (RF). Carotid artery was connected with pressure transducer for recording BP to determine the mean arterial pressure (MAP). ECG potentials were recorded by needle electrodes connected to bioamplifier using limb lead II configuration. All the recordings were made on a computerised chart recorder (Lab Chart 7, AD Instrument, Australia). Heart rate (HR) was calculated from R to R interval.[4,16]
Experimental design
After dissection and cannulation, the animals were allowed to stabilise for 30 min.[3] The rats were randomly divided into three groups.
In Group I (saline control group, n = 6), after initial recording, saline (75 μL) was injected through the jugular vein and the changes in RF, HR and BP were recorded at 2 min and then at every 15 min up to 120 min.[3] These animals served as time-matched control group.
In Group II (OA group, n = 6), after initial recording, 75 μL OA was injected through jugular vein and the changes in RF, HR and BP were recorded at 2 min and then at every 15 min up to 120 min or till the death of the animal, whichever was earlier.
In Group III (insulin + OA group, n = 6) after initial recording, insulin 0.2 unit (0.5 U/Kg of body weight) was injected intravenously through jugular vein and the changes in RF, HR and BP were recorded.[13] Fifteen minutes after insulin pre-treatment, OA 75 μL was injected and the recordings were taken as mentioned for Group II. At the end of each experiment, the lungs were excised. Lung of one side was kept for the estimation of pulmonary water content and other side was sent for histological examination.
Determination of pulmonary water content
The pulmonary water content was determined by physical method as described earlier.[2,4,17] Briefly, at the end of each experiment, the lungs were excised, one lung was preserved in formal saline for histological examination and the other was weighted and dried to a constant weight in an electric oven (at 90°C for 48 h). The difference between wet weight and dry weight was calculated to determine the water content.[4,17] This parameter was used as an indicator for pulmonary oedema developed during experiment.
PaO2/FiO2
The carotid arterial blood sample was collected into heparin rinsed syringe after 15 min of OA/saline injection in all groups.[16] The blood sample was subjected to arterial blood gas analysis (Roche OMNI gas analyser) to determine the PaO2/FiO2 ratio, where PaO2 is partial pressure of oxygen in arterial blood and FiO2 is fraction of oxygen in the inspired air (FiO2 considered as 0.21).
Histology of lungs
The lung tissue was fixed in formal saline and was subjected to standard histological protocol and stained with haematoxylin and eosin for microscopic examination.[18]
Drugs and solutions
Urethane was obtained from Sigma-Aldrich Inc., St. Louis, USA, and prepared in double-distilled water. OA was obtained from Hi-Media Laboratories Pvt. Limited, Mumbai. Insulin (2 IU) was obtained from Gland Pharma Ltd., Hyderabad.
Analysis of data
The changes in RF, HR and MAP were expressed as % of initial. The data were pooled and mean ± standard error of mean (SEM) were calculated. Sigma Plot 10.0 and MS Excel software were used for the statistical and graphical analysis. The comparisons among the groups were performed using one-way ANOVA (Kruskal–Wallis test) followed by post hoc test (Student’s Newman–Keuls test) to find out pairwise differences in mean values. Wilcoxon paired sample test was used to test significant changes at two time points that is, initial to 30 min within the groups. Kaplan–Meier’s survival curves and log rank (Mantel–Haenszel) test were used for comparing the survival time.[19] P < 0.05 was considered statistically significant.
RESULTS
Effect of saline on pulmonary and cardiac parameter
Saline-treated animals were taken as control group. After stabilisation, recording of RF, HR and BP was done. There was no significant change observed in the RF, HR and MAP [Figures 1-3] throughout the recording time (120 min) in saline treated rats. All the animals in this group survived for the entire observation period [Figure 4]. The PaO2/FiO2 was 454.67 ± 3.87 and pulmonary water content in this group was 77.74 ± 0.83% [Figure 5]. Histological examination of lungs exhibited the well-aerated alveoli which form the parenchyma of the lung. Alveoli were lined by a simple squamous epithelium [Figure 6a and b].

- Effect of OA (75 µl) on RF (% of initial) in rats without or with insulin pre-treatment. Insulin was injected 15 min before injection of OA. RF at ‘0’ min (time of OA injection) was taken as 100%. Mean ± standard error of mean values were obtained from six different experiments in control, OA and insulin + OA groups. Changes in the RF in insulin + OA group were significantly different from OA group (P<0.05; one-way ANOVA). Insulin + OA = Insulin + OA-treated group. OA: Oleic acid, RF: Respiratory frequency.
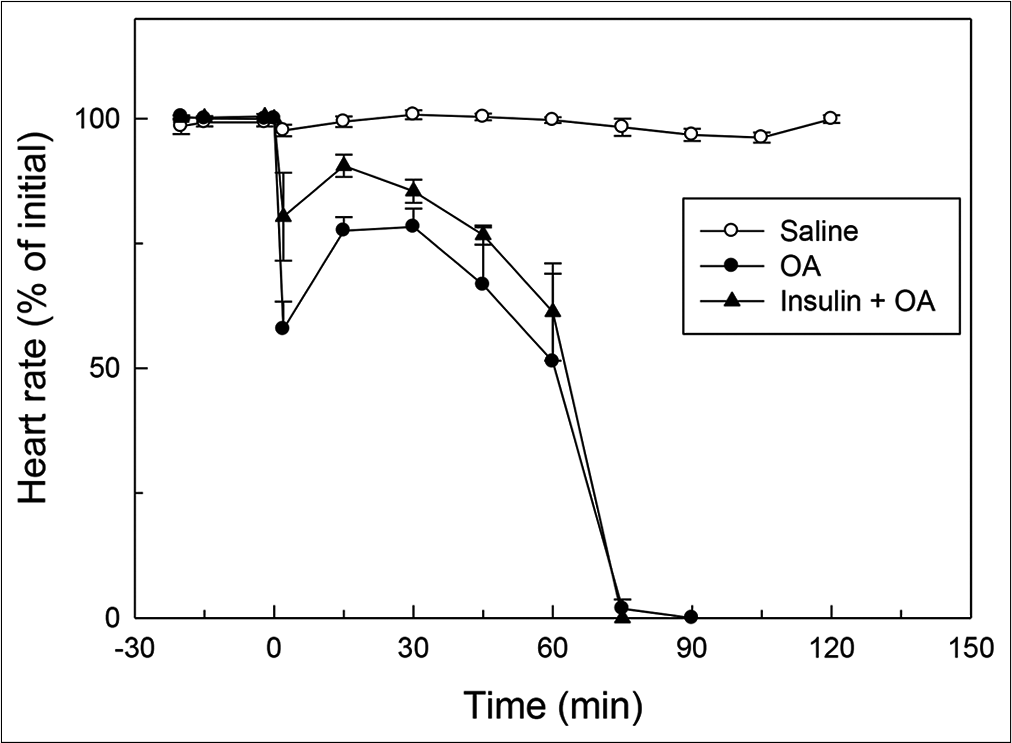
- Effect of OA (75 µL) on HR (% of initial) in rats without or with insulin pre-treatment. OA was injected at ‘0’ min. Insulin was injected 15 min before injection of OA. HR at ‘0’ min (time of injection of OA) was taken as 100%. Mean ± standard error of mean values were obtained from six different experiments in both insulin + OA group and in OA group. Changes in the insulin + OA group were similar to OA group (P<0.05; one-way ANOVA). Insulin + OA = Insulin + OA-treated group. OA: Oleic acid, HR: Heart rate.
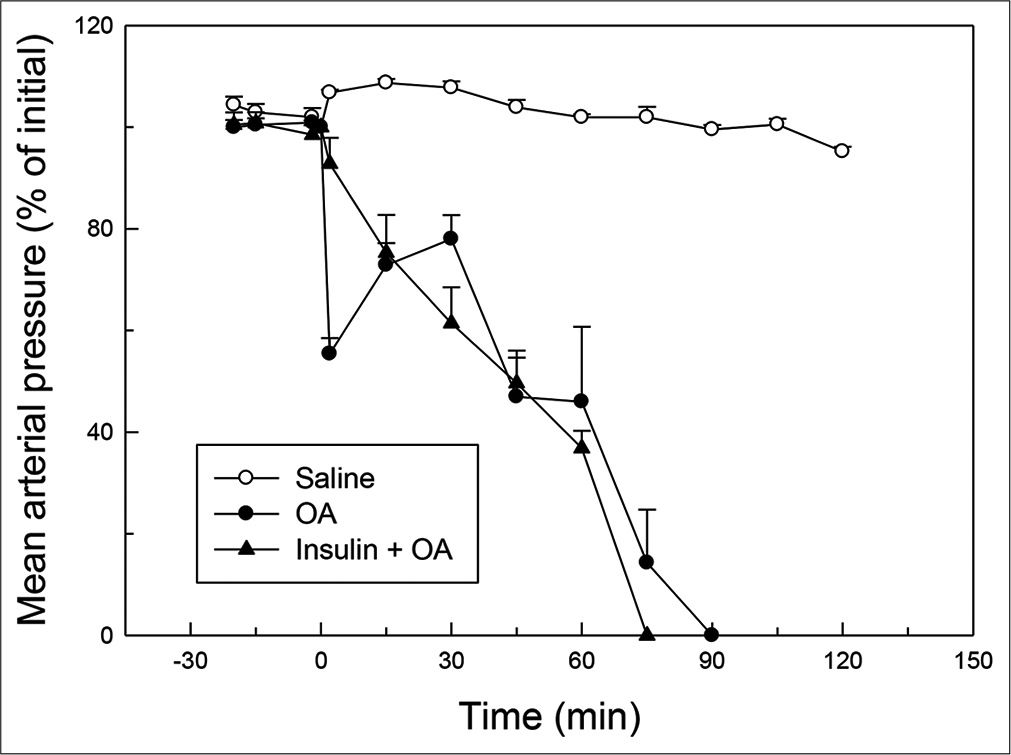
- Effect of OA (75 µL) on MAP (% of initial) in rats with or without insulin pre-treatment. OA was injected at ‘0’ min. Insulin was injected 15 min before injection of OA. MAP at ‘0’ min (time of injection of OA) was taken as 100%. Mean ± standard error of mean values were obtained from six different experiments in both insulin + OA group and in OA only group. Changes in the insulin + OA group were similar to OA group except at 2 min after OA injection (P<0.05; One-way ANOVA). Insulin + OA = Insulin + OA-treated group. OA: Oleic acid, MAP: Mean arterial pressure.
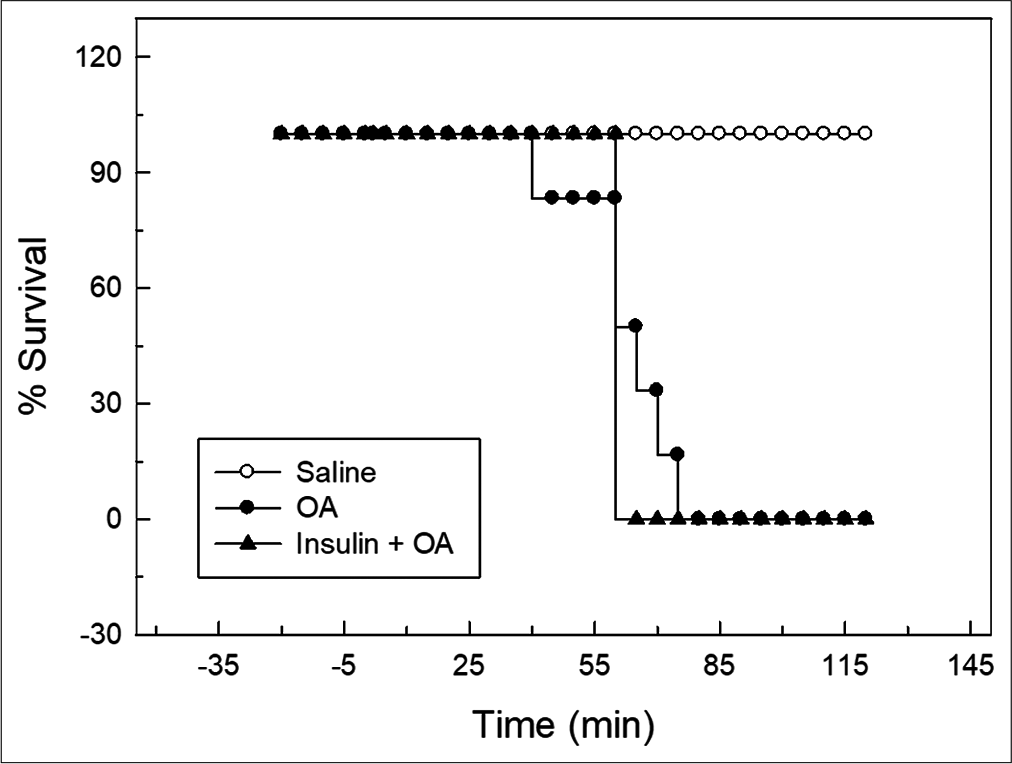
- Kaplan–Meier’s survival plot for control, OA and insulin + OA groups. Overall survival in insulin + OA group was similar to OA group. Insulin + OA = Insulin + OA treated group, OA = Oleic acid.
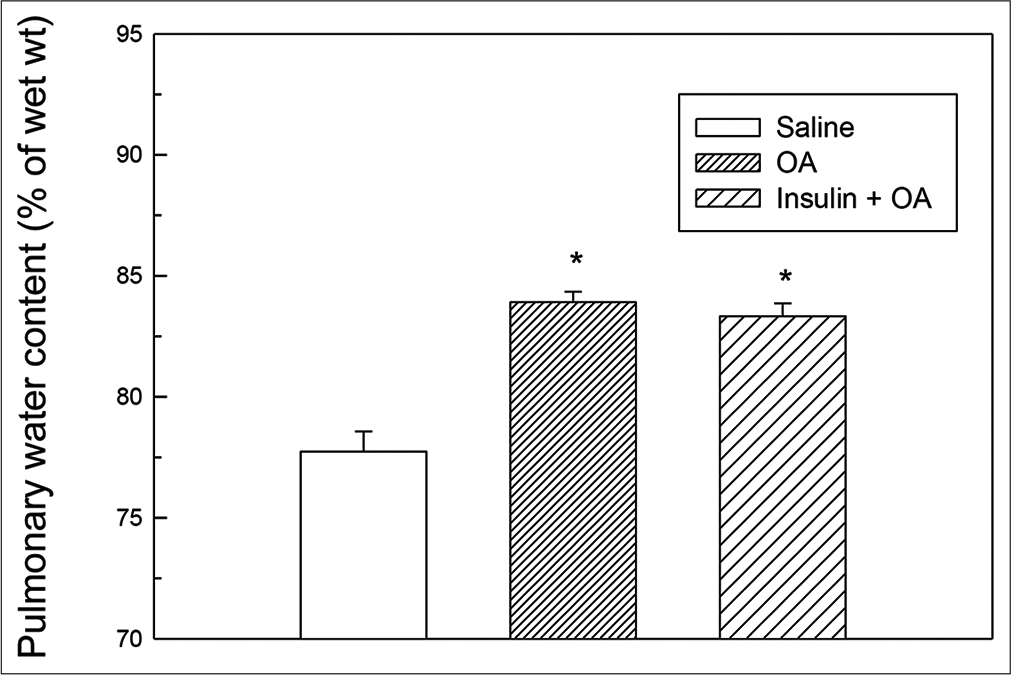
- Pulmonary water content (as % of wet lung tissue) in saline, OA and insulin + OA groups. Each bar depicts the Mean ± standard error of mean values obtained from six different experiments in each group. The asterisk (*) indicates significant difference from saline treated (control) group (P<0.05). Insulin + OA = Insulin + OA-treated group. OA: Oleic acid.
![Photomicrograph of rat lung in saline control (a and b); oleic acid treated (c and d); insulin + oleic acid (e and f) groups are shown. (Magnification = ×400. “↖” [thick arrow] depicts alveolar damage, “ϟ” depicts infiltration of inflammatory cells and “↘” [thin arrow] interalveolar septa, “red arrow” depicts endothelial lining of blood vessels and “yellow arrow” depicts intravascular congestion).](/content/114/2021/65/2/img/IJPP-65-103-g006.png)
- Photomicrograph of rat lung in saline control (a and b); oleic acid treated (c and d); insulin + oleic acid (e and f) groups are shown. (Magnification = ×400. “↖” [thick arrow] depicts alveolar damage, “ϟ” depicts infiltration of inflammatory cells and “↘” [thin arrow] interalveolar septa, “red arrow” depicts endothelial lining of blood vessels and “yellow arrow” depicts intravascular congestion).
OA produced ALI
In OA group, after OA (75 μL) administration, there was a significant increase in RF (twice the initial, P < 0.05) by 30 min followed by progressive fall in RF [Figure 1]. By 90 min, respiration stopped leading to death of all the animals. The mean survival time in this group was 69.5 ± 3.55 min [Figure 4]. OA administration produced an immediate decrease in HR (43% of initial, P < 0.05) and MAP (about 45% of initial, P < 0.05; Figures 2 and 3) followed by recovery (about 23% of initial) by 30 min and then progressive decrease till death of the animals [Figure 4]. The mean ± SEM of PaO2/FiO2 (250.40 ± 9.03) was significantly lesser (P < 0.05) and pulmonary water content (83.91 ± 0.44%) was significantly increased (P < 0.05) as compared to control group [Figure 5]. Histological examination of lungs showed multifocal and heterogeneous lung injury, with interstitial oedema and infiltration of leucocytes leading to thickening of the alveolar septa. Intravascular congestion and capillary endothelial injury were apparent. The entire lung architecture was destroyed in certain areas and the alveolar space was found to be reduced [Figure 6c and d].
Effect of insulin pre-treatment on OA-induced ALI
The initial tachypnoea, as observed in OA only group, was not seen in insulin pre-treated (insulin + OA) group. The RF was maintained at good level (92% of initial, P < 0.05) till 30 min [Figure 1]. Then, there was progressive fall in RF till death of the animal and mean survival time in this group of animals was 66.83 ± 1.28 min, similar to OA only group (Figure 4; Kaplan–Meier’s survival plot, log rank test, P= 0.14,). HR and MAP showed significant decrease (about 20%, P < 0.05) followed by recovery and then progressive decrease till death of the animal [Figures 2 and 3]. The mean ± SEM of PaO2/FiO2 was 275.86 ± 2.44 and pulmonary water content 83.33 ± 0.53%, respectively, and was similar to OA group (250.40 ± 9.03 and 83.91 ± 0.44%; Figure 5). Histological examination of lungs exhibited multifocal and heterogeneous injury in these rats also. However, inflammatory cells seen in interalveolar septa and alveoli were less in number (per field of histological slide examination) as compared to OA group. Thickening of the alveolar septa in this group was also less as compared to OA group. Intravascular congestion similar to OA group was seen in this group also but injury to the endothelial lining of blood capillaries was not observed in this group of animals [Figure 6 e and f].
DISCUSSION
ALI is a critical clinical condition characterised by hypoxemia, damage to the lung parenchyma, inflammation and development of pulmonary oedema.[1] As reported in our earlier studies in male albino rat, OA administration produced ALI in this study also.[3,4,16,18] Hypoxemia manifested as severe tachypnoea is found in the early stage of ALI. Damage to the lung parenchyma (epithelium/ endothelium) and inflammation was also observed in histological examination of lungs in these rats. OA administration produced pulmonary oedema in these rats ultimately leading to death. There were severe changes in the HR and MAP also. In this study, the rats pre-treated with insulin did not exhibit initial tachypnoea as seen in OA group. Further, histological examination of the lungs showed less number of inflammatory cells (per field of histological slide examination) in the interalveolar septa and alveoli and no endothelial damage as compared to OA group. However, insulin pre-treatment failed to alter the overall course of the disease as the pulmonary oedema and survival time was similar to OA group.
Insulin is a peptide hormone produced by the beta cells of the pancreatic islet and is considered to be an important anabolic hormone of the body. In human study elsewhere, it has been shown that intensive insulin therapy reduces morbidity and mortality among critically ill non-diabetic patients.[8] Although, the concern of ALI was not exclusively examined in these studies, it was observed that patient receiving intensive insulin therapy needed less mechanical ventilation.[8,9]
In other clinical studies of critical illness and animal studies, insulin has attenuated the systemic inflammatory response and is shown to be immunomodulatory through alteration in pro-inflammatory and anti-inflammatory cytokines and through mannose binding lectin pathway.[12,20,21] Earlier reports show also that insulin prevents endothelial dysfunction, neutrophil chemotaxis and leucocyte adhesion.[10,11,21] All these mechanisms have been implicated in the pathogenesis of ALI. Results of our study also support these observations as insulin pre-treatment prevented severe initial respiratory dysfunction and decreased the number of inflammatory cells in the lungs. In addition, insulin pre-treatment also prevented OA-induced endothelial damage which is well observed in the histological examination of the lungs. Although, in the present study, insulin failed to prevent the development of pulmonary oedema and death of the animals in ALI, it protected the animals in the initial phase of the disease and may be beneficial in this stage.
SUMMARY AND CONCLUSIONS
Insulin (an immunomodulatory agent) could not alter the course of the disease but may prevent the severe changes in the early stage of ALI. It reduces inflammation and endothelial damage seen in ALI. Further research is required to identify the role of insulin in ALI. A better understanding of the role of insulin in ALI will help in the diagnosis and management of this critical condition.
AUTHORS’ CONTRIBUTIONS
SK contributed in the data collection, analysis and interpretation of data, drafting of the initial draft of this article and subsequent final editing of this report. PB contributed in analysis and interpretation of data, drafting of the initial draft of this article and subsequent final editing of this report. SP contributed in data collection (Histology). RP was mentor, contributed to the conception of this work and final editing of this report.
Declaration of patient consent
Patient’s consent not required as there are no patients in this study.
Financial support and sponsorship
Nil.
Conflicts of interest
There are no conflicts of interest.
References
- Acute lung injury: Epidemiology, pathogenesis, and treatment. J Aerosol Med Pulm Drug Deliv. 2010;23:243-52.
- [CrossRef] [Google Scholar]
- An official American Thoracic Society workshop report: Features and measurements of experimental acute lung injury in animals. Am J Respir Cell Mol Biol. 2011;44:725-38.
- [CrossRef] [Google Scholar]
- Pheniramine maleate attenuates oleic acid-induced acute respiratory distress syndrome in rats. Indian J Exp Biol. 2017;55:351-6.
- [Google Scholar]
- Characterization of oleic acid-induced acute respiratory distress syndrome model in rat. Indian J Exp Biol. 2014;52:712-9.
- [Google Scholar]
- The acute respiratory distress syndrome. J Clin Invest. 2012;122:2731-40.
- [CrossRef] [Google Scholar]
- Acute lung injury and the acute respiratory distress syndrome: Four decades of inquiry into pathogenesis and rational management. Am J Respir Cell Mol Biol. 2005;33:319-27.
- [CrossRef] [Google Scholar]
- Intensive insulin therapy in critically ill patients. N Engl J Med. 2001;345:1359-67.
- [CrossRef] [Google Scholar]
- Intensive insulin therapy in the medical ICU. N Engl J Med. 2006;354:449-61.
- [CrossRef] [Google Scholar]
- Intensive insulin therapy protects the endothelium of critically ill patients. J Clin Invest. 2005;115:2277-86.
- [CrossRef] [Google Scholar]
- Insulin treatment improves the systemic inflammatory reaction to severe trauma. Ann Surg. 2004;239:553-60.
- [CrossRef] [Google Scholar]
- Intensive insulin therapy exerts antiinflammatory effects in critically ill patients and counteracts the adverse effect of low mannose-binding lectin levels. J Clin Endocrinol Metab. 2003;88:1082-8.
- [CrossRef] [Google Scholar]
- Insulin attenuates endotoxin-induced acute lung injury in conscious rats. Crit Care Med. 2006;34:758-64.
- [CrossRef] [Google Scholar]
- Captopril augments acetylcholine-induced bronchial smooth muscle contractions in vitro via kinin-dependent mechanisms. Indian J Exp Biol. 2016;54:365-9.
- [Google Scholar]
- Indomethacin exacerbates oleic acid-induced acute respiratory distress syndrome in adult rats. Indian J Physiol Pharmacol. 2016;60:82-9.
- [Google Scholar]
- Pulmonary oedema produced by scorpion venom augments a phenyldiguanide-induced reflex response in anaesthetized rats. J Physiol. 1999;521:537-44.
- [CrossRef] [Google Scholar]
- Protective role of prostaglandin E1 analog in indomethacin-induced deterioration in acute respiratory distress syndrome in rats. Natl J Physiol Pharm Pharmacol. 2017;7:749-53.
- [CrossRef] [Google Scholar]
- Understanding survival analysis: Kaplan-Meier estimate. Int J Ayurveda Res. 2010;1:274-8.
- [CrossRef] [Google Scholar]
- Diabetes, insulin, and development of acute lung injury. Crit Care Med. 2009;37:2455-64.
- [CrossRef] [Google Scholar]
- Modulation of the glycemic response using insulin attenuates the pulmonary response in an animal trauma model. J Trauma. 2007;63:351-7.
- [CrossRef] [Google Scholar]