Translate this page into:
Effect of oscillatory lower body negative pressure and isometric handgrip exercise on cardioautonomic tone of healthy individuals
*Corresponding author: Kishore K. Deepak, Department of Physiology, All India Institute of Medical Sciences (AIIMS), New Delhi, India. kkdeepak@gmail.com
-
Received: ,
Accepted: ,
How to cite this article: Banodhe GK, Sharma HB, Deepak KK. Effect of oscillatory lower body negative pressure and isometric handgrip exercise on cardioautonomic tone of healthy individuals. Indian J Physiol Pharmacol. 2024;68:18-24. doi: 10.25259/IJPP_553_2022
Abstract
Objectives:
The influence of the autonomic nervous system on the heart can be quantified by assessing changes in the heart rate variability (HRV) during orthostatic challenge and exercise. However, the combination of oscillatory lower body negative pressure (oLBNP) and isometric handgrip exercise (IHE) on HRV has not been previously investigated. Therefore, the present study aimed to assess the effects of (oLBNP) at sympathetic (0.1 Hz) and parasympathetic (0.25 Hz) frequencies and IHE at 30% of maximum voluntary contraction (MVC) on HRV in normal healthy individuals.
Materials and Methods:
We conducted a cross-sectional study on 18 healthy male subjects. Beat-to-beat blood pressure, lead-II electrocardiogram, and oLBNP chamber pressure were recorded continuously during oLBNP at 0.1 and 0.25 Hz for 3 min each and IHE at 30% of MVC (for 4 min) along with oLBNP at 0.1 or 0.25 Hz (oLBNP was added in last 3 min) each followed by HRV analysis.
Results:
The oLBNP at 0.25 Hz reduced the root mean square of successive inter-beat (RR) interval differences significantly as compared to baseline values and came to normal during the recovery phase (P = 0.008). The absolute power of the high-frequency band (HF power), Poincaré plot standard deviation perpendicular to the line of identity (SD1), and percentage of successive RR intervals that differ by more than 50 ms (pNN50) were also reduced significantly during oLBNP at 0.25 Hz and when IHE at 30% of MVC (IHE) was added to oLBNP at 0.25 Hz as compared to baseline (P < 0.05).
Conclusion:
oLBNP and IHE could be used as a non-invasive haemodynamic stressor to assess the neurocardiac axis and its mechanism of action during orthostatic stresses.
Keywords
Heart rate variability
Oscillatory lower body negative pressure
Isometric handgrip exercise
Maximum voluntary contraction
INTRODUCTION
Heart rate variability (HRV) is a physiological phenomenon, and it indicates the autonomic modulation of the heart rate (HR). The different HRV parameters correlate well with sympathetic and parasympathetic influences on the cardiovascular system. For example, root mean square of successive RR interval differences (RMSSD), SD1 (Poincare plot standard deviation perpendicular to the line of identity), high-frequency (HF) power and percentage of successive RR intervals that differ by more than 50 ms (pNN50) denotes more about parasympathetic influence and sympathetic influences can be evaluated with the help of low-frequency (LF) (Absolute power of the low-frequency band [0.04–0.15 Hz] and SD2 (Poincare plot standard deviation along the line of identity).[1]
Autonomic dysfunction led to a decrease in HRV due to an imbalance between the sympathetic and parasympathetic components of the autonomic nervous system. Hence, the HRV can be used as an assessment tool to detect early changes in the autonomic nervous system in asymptomatic individuals with systemic disorders associated with autonomic dysfunction.[2,3] HRV can also be used as a prognostic biomarker of metabolic disorders and cancer survival.[4]
Autonomic reactivity can help us understand the physiological basis of haemodynamic adjustments during various interventions such as exercise tests, orthostatic stress, lower body negative pressure (LBNP), and post-exercise venous occlusion. It may give us an insight into physical fitness.[5,6] The most common time domain variables used for the measurement of HRV are RMSSD and pNN50. The frequency domain variables frequently used are total power, HF, LF, and LF: HF ratio.[7,8] It has been seen that RMSSD (compute beat-to-beat variability) and standard deviation of R-R interval (SDNN, compute overall variability) reduce significantly during exercise, and the reduction is positively correlated with duration and intensity of exercise.[9] The reduction in RMSSD and SDNN values during exercise is due to enhanced sympathetic activity and vagal withdrawal.[10]
It has already been seen that HRV also reduces during LBNP application.[11] It is a fact that exercise therapy improves autonomic function and can be used in patients with systemic disorders to improve autonomic modulation.[12-15] As reported earlier (Weippert et al., 2013), the RMSSD decreased significantly during static exercise as compared to dynamic exercise.[16] HRV also shows a time-dependent recovery after the stoppage of exercise, and post-exercise recovery of HRV measures can be used as an assessment tool to evaluate the status of autonomic reactivity.[17] Hence, we used oscillatory LBNP (oLBNP) to oscillate blood pressure in low and high frequencies to see the effects of exercise on HRV at sympathetic and parasympathetic frequency bands.
The effect of exercise on HRV is variable and dependent on the type and intensity of exercise, and it has been seen that HRV tends to reduce with an increase in intensity of exercise.[18] Hence, the present study was done with the aim of assessing the effects of oLBNP at low (0.1 Hz) and high (0.25 Hz) frequencies and isometric handgrip exercise (IHE) at 30% of maximum voluntary contraction (MVC) on HRV. To the best of our knowledge, this is the first study to assess the effects of oLBNP and IHE on cardioautonomic tone in India.
MATERIALS AND METHODS
Subject and study protocol
Eighteen healthy male volunteers were included in the study. All subjects gave written informed consent before starting the study. The sample size was determined in line with the previous studies on LBNP and IHE.[18] The ethical clearance was taken from the Institute Ethics Committee, AIIMS, New Delhi (Reference No. IECPG-444/27.09.2018). The laboratory measurements were performed in the Autonomic and Vascular Function Laboratory, Department of Physiology, All India Institute of Medical Sciences, New Delhi. The subjects were asked not to take tea, coffee, and heavy breakfast four hours before the tests. The subject was asked to avoid physical exercise and alcohol 48 hours before the testing. All subjects were put in supine rest for 10 min after placing them in an oLBNP chamber with an airtight seal at the level of the iliac crest.
After their baseline recording, all subjects went through four interventions to assess HRV changes: (1) oLBNP at 0.1 Hz, (2) oLBNP at 0.25 Hz, (3) IHE with oLBNP at 0.1 Hz, and (4) IHE with oLBNP at 0.25 Hz and recovery was recorded for 10 min, as shown in Figure 1.
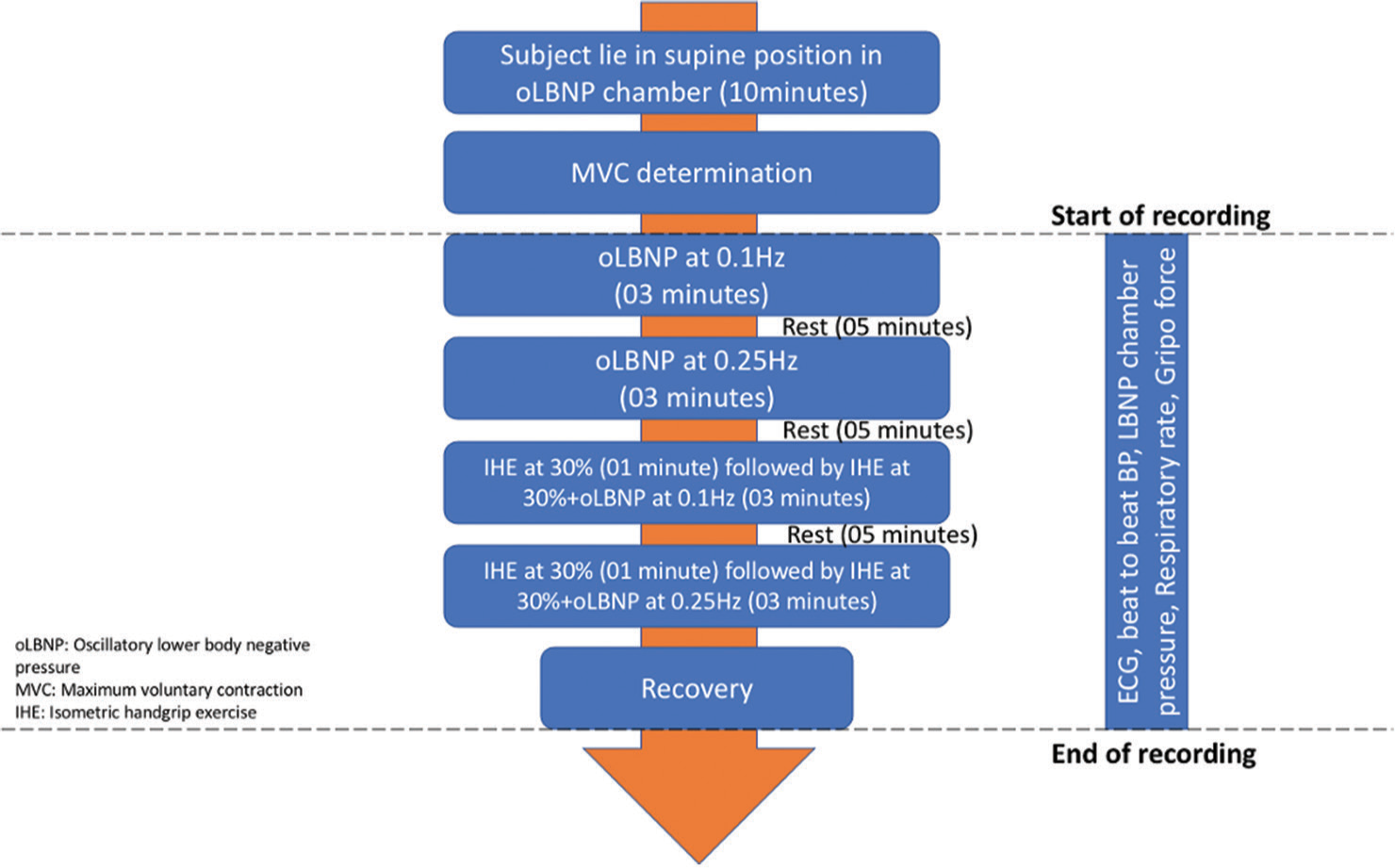
- Flow chart of the study protocol.
Resting ECG was used for the analysis of HRV after 10 min of supine rest. The ECG was recorded in lead II configuration using LabChart 8 (AD Instruments, Australia). After completion of the medical history and preliminary examination, the participants were asked to lie in a supine position inside the oLBNP chamber (VACUSPORT Regeneration System, Germany). The oLBNP chamber consists of a hollow cylindrical tube closed at one end and a curtain-seal at the other end. oLBNP chamber had a control panel outside it that could help the investigator set the intensity and duration of negative pressure. Their legs were sealed inside the chamber at the level of the iliac crest. Beat-to-beat BP was recorded using the Human Non-Invasive BP System (Human NIBP; Finapres Medical Systems, The Netherlands), and an oscillatory suction pressure (maximum pressure was 40 mmHg) was generated at 0.1 Hz and 0.25 Hz frequencies for 3 min followed by 5 min of rest. After the determination of the MVC of subjects, they were asked to do IHE at 30% of MVC (IHE) with the help of a grip force transducer (MLT/004 ST) for 4 min, and during the last 3 min of IHE, oLBNP at 0.1 Hz was also added. A similar recording was done for IHE and 0.25Hz oLBNP. Beat-to-beat blood pressure (Human NIBP System, Finapres Medical Systems, Netherlands), respiratory rate (TN1132/ST), and oLBNP chamber pressure (MLT1199 BP transducer) were monitored continuously during the protocol.
HRV analysis
ECG recording was obtained using Lab Chart 8 (AD Instruments, Australia). R-peaks were detected automatically after manually selecting the segment of ECG intended for HRV analysis. For HRV analysis, R-R interval and histogram bin width were kept at 800–1200 ms and 10 ms, respectively. The ECG recording was checked visually to detect any missed R-peak and the presence of artefacts. After processing the ECG signal, time-domain and frequency-domain analysis were done by statistical analysis of the R-R interval and Lomb Periodogram, respectively, by the HRV software module automatically in Lab Chart 8. The Lomb periodogram is a mathematical algorithm for identifying and assessing the periodicity in unevenly distributed time-series data. It has been widely used to compute the Fourier-like power spectrum, leading to the determination of the approximate period of oscillation of unevenly distributed time-series data like ECG signals.[19]
Statistical analysis
The data were analysed using Statistical Package for the Social Sciences (SPSS) version 20. The normality of the data was assessed by performing the Shapiro–Wilk test. Repeated measure comparison of the studied parameters was done using Friedman’s test, and then the Bonferroni post hoc test was applied. Statistical significance was set at P ≤ 0.05.
RESULTS
HRV parameters used for sympathetic activity were absolute power of the low-frequency band (0.04–0.15 Hz) (LF power [ms2]) and Poincaré plot standard deviation along the line of identity (SD2 [ms]). HRV parameters used for parasympathetic activity were total power (TP, ms2), root mean square of successive RR interval differences (RMSSD, ms), standard deviation of the differences between successive NN intervals (SDSD, ms), absolute power of the HF band (0.15–0.4 Hz) (HF, ms2), Poincaré plot standard deviation perpendicular the line of identity {SD1(ms)}, standard deviation of all NN intervals (SDNN, ms) and percentage of successive RR intervals that differ by more than 50 ms (pNN50,%). HRV parameters for sympathovagal control used were the ratio of LF-to-HF power (LF/HF, %) and the ratio of SD1-to-SD2 (SD1/SD2, %).
Effect of oLBNP on HRV
The HRV parameters, which denote sympathetic, parasympathetic, and sympathovagal control, did not change at the application of low frequency (0.1 Hz) oLBNP as compared to baseline. Still, during the application of a high-frequency oLBNP (0.25 Hz), the markers of parasympathetic control, that is, RMSSD (P = 0.001), SD1 (P = 0.001) and pNN50 (P < 0.001) were significantly reduced, as shown in Tables 1 and 2. The HRV parameters such as HF power (P = 0.022), SDSD (P < 0.001), and SD1/SD2 (P < 0.001) were also significantly low during high-frequency oLBNP application as compared to low-frequency OLBNP, as shown in Tables 2 and 3.
HRV parameters: Markers of sympathetic activity | |||||||
---|---|---|---|---|---|---|---|
S.No. | Experimental conditions: Median±QD | ||||||
A | B | C | D | E | F | P-value | |
Baseline | 0.1Hz oLBNP | 0.25HZ oLBNP | MVC30% +0.1Hz oLBNP | MVC30% +0.25Hz oLBNP | Recovery | ||
1. LF (ms2) | 873.2±306.08 | 1091.5±472.75 | 754.85±245.46 | 1622±515.63 | 1263.5±785.90 | 1239.5±828.30 | 0.227a, 0.709b, 0.008*c, 0.079d, 0.053e |
2. SD2 (ms) | 65.47±8.16 | 68.85±18.55 | 68.08±15.32 | 77.44±15.02 | 81.49±14.55 | 83.78±9.55 | 0.318a, 0.205b, 0.087c, 0.072d, 0.004*e |
Median±QD; QD: Quartile deviation. Friedman’s Test.*P<0.05.; MVC: Maximum voluntary contraction, oLBNP: Oscillatory lower body negative pressure, aComparison between column A and B of the same row, bComparison between column A and C of the same row, cComparison between column A and D of same row, dComparison between column A and E of the same row, eComparison between column A and F of same row. SD: Standard deviation, LF: Low-frequency, HRV: Heart rate variability, IHE: Isometric handgrip exercise
HRV parameters: markers of parasympathetic activity | |||||||
---|---|---|---|---|---|---|---|
S.No. | Experimental conditions: Median±QD | ||||||
A | B | C | D | E | F | P-value | |
Baseline | 0.1 Hz oLBNP | 0.25 Hz oLBNP | MVC30% +0.1Hz oLBNP |
MVC30% +0.25Hz oLBNP |
Recovery | ||
1. Total Power (TP) (ms2) | 2158±601.62 | 2793.50±1291.50 | 2134.50±1041.62 | 3596.50±769.75 | 3221±1581.37 | 3822.5±1737.75 | 0.466a, 0.784b, 0.275c, 0.412d, 0.012*e |
2. RMSSD (ms) | 44.755±9.05 | 44.09±8.87 | 31.15±5.55 | 37.11±12.86 | 26.36±10.62 | 47.81±10.58 | 0.960a, 0.001*b, 1.000c, 0.0.058d, 0.099e, 0.001*f, 0.058g, |
3. SDSD (ms) | 44.815±9.07 | 44.19±8.90 | 31.215±5.57 | 37.185±12.89 | 26.42±10.67 | 47.9±10.55 | 1.000a, 0.001*b, 1.000c, 0.045*d 0.087e,<0.001*f |
5. HF (ms2) | 770.15±429.31 | 804.75±345.36 | 439.2±214.50 | 570.6±407.05 | 294.65±180.78 | 975.9±623.59 | 0.440a, 0.003*b, 0.248c, 0.010*d 0.499e, 0.022*f, 0.150g |
7. SD1(ms) | 31.69±6.41 | 31.25±6.30 | 22.07±3.93 | 26.29±9.12 | 18.69±7.54 | 33.87±7.46 | 1.000a, 0.001*b, 1.000c, 0.045*d 0.087e, 0.001*f, 0.045*g |
9. SDNN (ms) | 52.16±6.02 | 53.77±12.51 | 51.69±10.19 | 59.54±10.88 | 61.66±12.25 | 63.66±8.03 | 0.587a, 0.856b, 0.207c, 0.366d 0.013*e |
10. pNN50 (%) | 23.91±11.61 | 21.30±9.63 | 9.68±5.78 | 14.68±7.68 | 6.13±6.96 | 26.11±13.50 | 0.596a, <0.001*b, 0.140c, <0.001*d, 0.525e, <0.001*f, 0.036*g |
Median±QD); QD: Quartile deviation. Friedman’s Test.*P<0.05.; MVC: Maximum voluntary contraction, oLBNP: Oscillatory lower body negative pressure, aComparison between column A and B of the same row, bComparison between column A and C of the same row, cComparison between column A and D of same row, dComparison between column A and E of same row, eComparison between column A and F of same row, fComparison between column B and C of the same row, gComparison between column D and E of same ro. SD: Standard deviation, LF: Low-frequency, HRV: Heart rate variability, HF: High-frequency, IHE: Isometric handgrip exercise, RMSSD: Root mean square of successive RR interval differences, SDNN: Standard deviation of R-R interval, pNN: Percentage of successive RR intervals that differ by more than 50ms, SDSD: Standard deviation of the differences between successive NN intervals
HRV parameters: markers of sympathovagal balance | |||||||
---|---|---|---|---|---|---|---|
S. No. | Experimental conditions: Median±QD | ||||||
A | B | C | D | E | F | P-value | |
Baseline | 0.1 Hz oLBNP | 0.25 Hz oLBNP | MVC30% +0.1 Hz oLBNP | MVC30% +0.25 Hz oLBNP | Recovery | ||
1. LF/HF (%) | 0.84±0.78 | 1.50±0.45 | 1.46±0.36 | 2.17±1.61 | 3.25±1.09 | 1.13±0.43 | 0.140a, 0.291b, <0.001*c, <0.001*d, 1.000e |
2. SD1/SD2 (%) | 0.500±0.133 | 0.431±0.095 | 0.329±0.072 | 0.371±0.066 | 0.332±0.056 | 0.433±0.098 | 0.195a, <0.001*b, <0.001*c, <0.001*d, 0.027e, <0.001*f |
Median±QD); QD: Quartile deviation. Friedman’s Test.*P<0.05.; MVC: Maximum voluntary contraction, oLBNP: Oscillatory lower body negative pressure, aComparison between column A and B of the same row, bComparison between column A and C of the same row, cComparison between column A and D of the same row, dComparison between column A and E of the same row, eComparison between column A and F of the same row, fComparison between column B and C of the same row. SD: Standard deviation, LF: Low-frequency, HRV: Heart rate variability, HF: High-frequency, IHE: Isometric handgrip exercise
Effect of IHE along with oLBNP on HRV
The markers of sympathetic nervous system activity, that is, LF power (P = 0.008); and sympathovagal balance, that is, LF/HF ratio (P < 0.001) increased significantly as compared to baseline when IHE was added to 0.1 Hz oLBNP, as shown in Tables 1 and 3, although markers of parasympathetic activity did not change much at 0.1 Hz oLBNP as compared to baseline. However, when IHE was added to 0.25 Hz oLBNP the markers of parasympathetic activity, that is, HF (P = 0.010), SD1 (P = 0.045) and pNN50 (P < 0.001) also reduced as compared to baseline, as shown in Table 2. RMSSD was also reduced at IHE+0.25 Hz oLBNP, but it was not statistically significant.
DISCUSSION
The present study comprehensively assesses autonomic reactivity, and the main finding of the present study is that HRV was significantly reduced during oLBNP and oLBNP with IHE at high frequency. The most commonly used parasympathetic parameters to show changes in HRV with different interventions are RMSSD, SD1, and pNN50 because they represent vagally-mediated HRV and are less affected by respiration. LBNP models have been used widely to simulate haemorrhage and evaluate the autonomic reactivity of patients by assessing their tendency to go into syncope during orthostatic stress.[11] HR oscillations were studied to understand the autonomic modulation of the heart in different frequencies.[19] Similarly, oLBNP models can be used to oscillate arterial blood pressure at sympathetic and parasympathetic frequencies to assess autonomic modulation of the heart by evaluating the changes in HRV parameters.
We found a significant reduction in HRV measures during oLBNP at a higher frequency oscillation (0.25 Hz). It has already been noted in the previous study that HRV tends to reduce during LBNP application.[11] The decrease in LF power during high-frequency oLBNP, although not statistically significant, was similar to an animal haemorrhage model. [20] The most probable reason for the significant reduction of RMSSD, SD1, and pNN50 during the application of 0.25 Hz oLBNP is a generation of sustained suction pressure of 40 mmHg, and this led to the pooling of blood in the lower limb, causing increased sympathetic activity due to decreased stroke volume and cardiac output[11] which initiated a compensatory response to normalise the stroke volume and cardiac output by increasing the sympathetic tone and cardiac contractility.[11,21]
We found a significant increment in LF during IHE with 0.1 Hz oLBNP as compared to the baseline, which denotes sympathetic activation during exercise. Similar results were also found by Kotwal et al., on prehypertensive young adults. They reported a significant increase in LF/HF ratio (P < 0.001) during isometric exercise in prehypertensive young adults.[18] Although, Gerage et al., (2013) did not find any significant change in HRV after resistance training in postmenopausal women.[22] The sympathetic activation plays a crucial role during exercise by increasing HR, blood pressure, and cardiac output to increase blood flow to the exercising muscle.[23] The central command and activation of the sympathetic system also led to parasympathetic withdrawal,[24] which can be seen as a decrease in HF, SD1, and pNN50 during IHE and 0.25 Hz oLBNP. We also reported an increase in the LF/HF ratio and a reduction in the SD1/SD2 ratio during IHE and oLBNP application, which clearly indicate the tilting of sympathovagal balance towards the sympathetic nervous system due to increased sympathetic outflow and vagal withdrawal.
The increase in sympathetic activation during static exercise[25] and reduction in parasympathetic activity have been reported earlier.[20] The possible mechanisms for this increase in sympathetic activity but the reduction in parasympathetic activity during IHE are activation of central command, increase in sympathetic modulation due to muscle metaboreflex,[26] and reduced vagal modulation of the SA node.[27-29] The sympathetic activity was still high (SD2recovery >SD1baseline) after cessation of IHE, but simultaneously parasympathetic reactivation (TPrecovery>TPbaseline) also occurred during the recovery phase, which is in line with the previous studies.[30] This shows that complete recovery might not have happened, and autonomic system recovery after IHE may take up to several hours.
These findings indicated the relationship between an autonomic system and isometric exercise, namely the IHE. Irrespective of the frequency (0.1 or 0.25 Hz), the addition of IHE led to sympathetic activation and parasympathetic reduction. Our findings provided the quantification of the sympathovagal balance during IHE irrespective of the frequency of oLBNP using HRV parameters such as LF/HF and SD1/SD2 ratio.
Limitations
Our study provides evidence showing changes in HRV during the application of oLBNP and IHE in sympathetic and parasympathetic frequency bands. One of the limitation of our study is a small sample size. Future studies with larger sample sizes are needed to substantiate the findings of the present study.
CONCLUSION
We conclude that the HRV reduces during IHE and oscillatory lower body negative application. Exercise acts as a stressor and helps in improving HRV during rest, which has been proved by a significant increase in HRV during a recovery phase. Similar changes were also seen during high-frequency oLBNP intervention. Together, these findings support the use of IHE and oLBNP for assessing cardiac autonomic tone in healthy young individuals and as a treatment modality to improve HRV in patients with autonomic dysfunction.
Ethical approval
The author(s) declare that they have taken the ethical approval from IEC (Reference No. IECPG-444/27.09.2018).
Declaration of patient consent
The authors certify that they have obtained all appropriate patient consent.
Conflicts of interest
There are no conflicts of interest.
Use of artificial intelligence (AI)-assisted technology for manuscript preparation
The authors confirm that there was no use of artificial intelligence (AI)-assisted technology for assisting in the writing or editing of the manuscript, and no images were manipulated using AI.
Financial support and sponsorship
Nil.
References
- Heart rate variability. Standards of measurement, physiological interpretation, and clinical use. Task Force of the European Society of Cardiology and the North American Society of Pacing and Electrophysiology. Eur Heart J. 1996;17:354-81.
- [CrossRef] [Google Scholar]
- Autonomic dysfunction in patients with fibromyalgia: Application of power spectral analysis of heart rate variability. Semin Arthritis Rheum. 2000;29:217-27.
- [CrossRef] [Google Scholar]
- Role of heart rate variability in the early diagnosis of diabetic autonomic neuropathy in children. Herz. 2002;27:785-90.
- [CrossRef] [Google Scholar]
- Heart Rate variability as a prognostic factor for cancer survival-a systematic review. Front Physiol. 2018;9:623.
- [CrossRef] [Google Scholar]
- Cardiac autonomic responses at onset of exercise: Effects of aerobic fitness. Int J Sports Med. 2014;35:879-85.
- [CrossRef] [Google Scholar]
- Impact of a soccer match on the cardiac autonomic control of referees. Eur J Appl Physiol. 2012;112:2233-42.
- [CrossRef] [Google Scholar]
- Kubios HRV--heart rate variability analysis software. Comput Methods Programs Biomed. 2014;113:210-20.
- [CrossRef] [Google Scholar]
- Methodological aspects of heart rate variability analysis. Heart. Available from: https://www.taylorfrancis.com/chapters/edit/10.1201/b12756-7/methodological-aspects-heart-rate-variability-analysis-tom-kuusela [Last accessed on 2022 Nov 17]
- [Google Scholar]
- Changes in heart rate variability with respect to exercise intensity and time during treadmill running. Biomed Eng Online. 2018;17:128.
- [CrossRef] [Google Scholar]
- Autonomic neural control of heart rate during dynamic exercise: Revisited. J Physiol. 2014;592:2491-500.
- [CrossRef] [Google Scholar]
- Heart rate variability analysis under lower body negative pressure. Space Med Med Eng (Beijing). 1998;11:430-4.
- [Google Scholar]
- Neural regulation of heart rate variability in endurance athletes and sedentary controls. Cardiovasc Res. 1992;26:713-9.
- [CrossRef] [Google Scholar]
- Early and late effects of exercise and athletic training on neural mechanisms controlling heart rate. Cardiovasc Res. 1993;27:482-8.
- [CrossRef] [Google Scholar]
- Interval training in elderly men increases both heart rate variability and baroreflex activity. Clin Auton Res. 2005;15:107-15.
- [CrossRef] [PubMed] [Google Scholar]
- Effects of cardiac rehabilitation and beta-blocker therapy on heart rate variability after first acute myocardial infarction. Am J Cardiol. 1998;81:834-40.
- [CrossRef] [PubMed] [Google Scholar]
- Heart rate variability and blood pressure during dynamic and static exercise at similar heart rate levels. PLoS One. 2013;8:e83690.
- [CrossRef] [PubMed] [Google Scholar]
- Effect of static and dynamic exercise on heart rate and blood pressure variabilities. Med Sci Sports Exerc. 2000;32:1719-28.
- [CrossRef] [PubMed] [Google Scholar]
- Effect of isometric exercise on heart rate variability in prehypertensive young adults. Med J Babylon. 2022;19:275.
- [CrossRef] [Google Scholar]
- Understanding the lomb-scargle periodogram. Astrophys J Suppl Ser. 2018;236:16.
- [CrossRef] [Google Scholar]
- Tilt testing with combined lower body negative pressure: A “gold standard” for measuring orthostatic tolerance. J Vis Exp. 2013;73:4315.
- [CrossRef] [Google Scholar]
- High-frequency autonomic modulation: A new model for analysis of autonomic cardiac control. Br J Pharmacol. 2018;175:3131-43.
- [CrossRef] [PubMed] [Google Scholar]
- Cardiovascular adaptations to resistance training in elderly postmenopausal women. Int J Sports Med. 2013;34:806-13.
- [CrossRef] [PubMed] [Google Scholar]
- Heart rate variability during simulated hemorrhage with lower body negative pressure in high and low tolerant subjects. Front Physiol. 2011;2:85.
- [CrossRef] [PubMed] [Google Scholar]
- Cardiovascular adaptations to resistance training in elderly postmenopausal women. Int J Sports Med. 2013;34:806-13.
- [CrossRef] [PubMed] [Google Scholar]
- Neural control of muscle blood flow during exercise. J Appl Physiol (1985). 2004;97:731-8.
- [CrossRef] [PubMed] [Google Scholar]
- Central command and the regulation of exercise heart rate response in heart failure with preserved ejection fraction. Circulation. 2021;143:783-9.
- [CrossRef] [PubMed] [Google Scholar]
- Reflex sympathetic activation during static exercise is severely impaired in patients with myophosphorylase deficiency. J Physiol. 2003;548:983-93.
- [CrossRef] [PubMed] [Google Scholar]
- Cardiac autonomic modulation adjustments in isometric exercise. MedicalExpress. 2015;2:M150102.
- [CrossRef] [Google Scholar]
- Reflex control of the circulation during exercise: Chemoreflexes and mechanoreflexes. J Appl Physiol(1985). 1990;69:407-18.
- [CrossRef] [PubMed] [Google Scholar]