Translate this page into:
Intermittent hypoxia exposure at sea level improves functional capacity (6MWT) at high altitude
*Corresponding author: Gopinath Bhaumik, Department of Applied Physiology, Defense Institute of Physiology and Allied Science, Defence Research and Development Organisation, New Delhi, Delhi, India. g_bhaumik1@yahoo.co.in
-
Received: ,
Accepted: ,
How to cite this article: Newmei MK, Reddy MP, Dass D, Singh V, Naik S, Bhaumik G. Intermittent hypoxia exposure at sea level improves functional capacity (6MWT) at high altitude. Indian J Physiol Pharmacol. 2023;67:290-5. doi: 10.25259/IJPP_579_2022
Abstract
Objectives:
Our primary objective is to observe whether acclimatisation is elicited by the intermittent hypoxic exposure (IHE) protocol. For this, we have utilised performance in a 6-min walk test (6MWT) as a tool to assess physiological responses to high altitude (HA) both in control and IHE-exposed groups, respectively.
Materials and Methods:
The study was a prospective cohort study conducted on Indian army volunteers (n = 57) and they were divided into two groups, a control group (CG) and an experimental group (EG). At the sea level, a baseline study was carried out on barometric pressure. IHE was performed at sea level in the normobaric hypoxia chamber (low fraction of inspired oxygen [FiO2], at normal barometric pressure, 740 mmHg), in which the FiO2 of the chamber was artificially decreased using O2-filtering membranes. The oxygen percentage was constantly maintained at 12%-13%. After recording the baseline, the subjects were exposed to a normobaric hypoxia chamber at 12%-13% FiO2 (altitude – equivalent to 4350 m Approx). Heart rate and blood pressure (BP) were recorded with a battery-operated portable BP monitor (OMRON) at both locations. A finger pulse oximeter probe was set on the right index finger to measure the resting oxygen saturation (SpO2) level (Model MU 300). Incidence of acute mountain sickness (AMS) was scored with the help of the standard Lake Louise questionnaire (LLS). Total LLS scores more than >3 (range 0–15) were considered AMS.
Results:
EG individuals that went through IHE performed better at 6MWT at Stage I (P = 0.03). EG also had better SpO2, levels as compared to CG (P = 0.00) at Stage II (P = 0.03). Furthermore, there was a significant difference in the Borg’s Scale between CG and EG. The Delta SpO2 of EG was better as compared to CG in all stages, albeit not significant (P = 0.07). There was a significant difference between IHE and CG groups, and CG was at an increased risk for lower SpO2 (8.00 [1.21–52.60], P = 0.03).
Conclusion:
The findings elucidate the benefits of IHE in rapid acclimatisation, and it contributed to better distance covered as shown by 6MWT as well and reduces hypoxic incidents in HA.
Keywords
Intermittent hypoxia exposure
6-min walk test
High altitude
Oxygen saturation
INTRODUCTION
One of the main environmental conditions at high altitude (HA) is the lowered atmospheric pressure of air, consequently, the partial pressure of oxygen (PO2) in the air decreases.[1] This lessening of the availability of oxygen in the blood prompts physiological changes.[2] This decreased PO2 is the major cause of altitude illnesses, with given time the body can acclimatise to the decrease in oxygen at a specific altitude.[3] There is a spectrum of cerebral and pulmonary syndromes that can manifest in unacclimatised persons after ascent to HA; the key ones are acute mountain sickness (AMS), HA pulmonary oedema, and HA cerebral oedema.[4] Acetazolamide is the preferred pharmacological drug generally used in the prevention of AMS.[5,6] However, an upcoming and alternative approach to induce acclimatisation at sea level is the use of intermittent hypoxic exposure (IHE).[7] IHE can be described as periodic exposure to hypoxia interrupted by a return to normoxia or less hypoxic conditions; this exposure can last for minutes or days.[8] Simulated altitude (both hypobaric and normobaric hypoxia) has been explored in sports science[9] for therapeutic purposes[10,11] and for pre-acclimatisation to HA.[12-15] A recent study from this laboratory on IHE indicated that normobaric hypoxic exposure at sea level significantly reduces AMS during subsequent exposure to hypobaric hypoxia at 3500 m altitude.[7,16] The 6-min walk test (6MWT) has been widely used in clinical and research settings to determine and monitor functional capacity.[17] 6MWT provides a better reflection of everyday activities compared to alternative walking assessments.[18] 6MWT is relatively low-cost, reproducible, and does not require sophisticated equipment, making it ideal for field studies. In some studies, related to HA, it has been used as a single measurement to ascertain changes in functional capacity amongst lowlanders at various altitudes, as well as a method to predict susceptibility to altitude sickness.[19,20]
Our primary objective is to observe whether preacclimatisation is elicited by the IHE protocol at sea level. We have employed 6MWT as a tool to evaluate the physiological responses to HA in both the control group (CG) and the experimental group (EG) to the IHE protocol, respectively.
MATERIALS AND METHODS
The study was a prospective cohort study conducted on all male volunteers (n = 57). The mean ± standard deviation (SD) values of age, height, and weight were 25.61 ± 6.45, 175.47 ± 4.02, and 69.64 ± 8.91, respectively, for the CG (n = 29). In the experimental group (EG) (n = 28) the mean ± SD value of age, height, and weight was 22.68 ± 2.76, 172.52 ± 4.54, and 66.08 ± 8.56, respectively. All the subjects were sea-level residents. None of the participants had been exposed to HA within the last month. The subjects were in good health with no evidence of cardiovascular or pulmonary diseases. The demographic characteristic is shown in [Table 1]. The study protocol was approved by the institute’s ethical committee, and informed consent from all the subjects was obtained and made aware of their right to withdraw at any time. At the sea level [Table 2], a baseline study was carried out at sea level (barometric pressure 740 mmHg). After recording the baseline, the subjects were exposed to a normobaric hypoxia chamber at 12–13% fraction of inspired oxygen (FiO2) (altitude – equivalent 4350 m) for 4 h/day for 4 consecutive days. On the 5th day, the subjects were inducted (within 24 h) to an altitude of approximately 3200 m at Stage I (barometric pressure 510 mmHg) in the Eastern Himalayas by road. During the period of study at this altitude, the ambient temperature varied between 2°C and 20°C, and relative humidity was around 40%. The tests were conducted in the morning hours for 6 consecutive days. The subjects were then moved to a higher altitude, Stage -II (4000 m, barometric pressure 460 mmHg) at a proximal location by road (1–2 h). The ambient temperature at this altitude varied between −2°C and 17°C. At this altitude, similar tests were carried out in the morning hours for 4 consecutive days. Heart rate (HR) and blood pressure (BP) were recorded with a battery-operated portable BP monitor (OMRON) at both locations. A finger pulse oximeter probe was set on the right index finger to measure the resting oxygen saturation (SpO2) level (Model MU 300). The incidence of AMS was scored with the help of the standard Lake Louise questionnaire (LLS).[21] Total LLS scores more than >3 (range 0–15) were considered AMS.[21] Individuals with SpO2 < 90% are considered hypoxic.[22,23] In this study, we adopted the threshold of SpO2 < 90% as an indicator of abnormal SpO, whereas SpO2 levels ≥ 90% were categorised as normal, in accordance with previously documented research.[22,23] We employed this methodology to facilitate odds ratio (OR) analysis within the context of IHE.
Variables | CG | EG |
---|---|---|
Age | 25.61±6.45 | 22.68±2.76 |
Weight | 69.64±8.91 | 66.08±8.56 |
Height | 175.47±4.02 | 172.52±4.54 |
BMI | 22.59±2.57 | 22.16±2.43 |
N | 29 | 28 |
CG: Control group, EG: Experimental group, BMI: Body mass index, N: Number of male volunteer
Variables | EG | CG | ||
---|---|---|---|---|
At rest (±SD), AR1 | 6MWT (±SD), E1 | At rest (±SD), AR2 | 6MWT (±SD), C2 | |
SpO2(%) | 98.45 (0.50) | 97.86 (0.69) | 98.03 (0.42) | 97.50 (0.56) |
HR (bpm) | 74.79 (9.33) | 87.07 (17.39) | 77.35 (10.91) | 86.89 (12.7) |
SBP (mmHg) | 116.21 (11.05) | 143.03 (16.19) | 121.07 (13.30) | 140.71 (15.1) |
DBP (mmHg) | 66.07 (0.50) | 82.03 (6.66) | 71.64 (11.77) | 81.25 (9.71) |
Borg’s scale | 0.60 (0.50) | 0.58 (0.91) | ||
6MWT (meter) | 686.83 (62.91) | 631.82 (30.71) | ||
Delta of SpO2(%) | 0.59 (0.56) | 0.53 (0.49) |
n=29 (EG) and n=28 (CG), AR1: At-rest experimental group, AR2: At-rest control group, E1: Experimental group after 6MWT, C2: Control group after 6MWT, EG: Experimental group, CG: Control group, 6MWT: 6-min walk test, SpO2: Oxygen saturation, HR: Heart rate, DBP: Diastolic blood pressure, SBP: Systolic blood pressure, SD: Standard deviation
IHE
IHE was performed at sea level in the normobaric hypoxia chamber (low FiO2, at normal barometric pressure), in which the FiO2 of the chamber was artificially decreased using O2-filtering membranes. The oxygen percentage was constantly maintained at 12–13%. Carbon dioxide was automatically scrubbed out and did not exceed 0.1% throughout the exposure. The chamber temperature was maintained between 20°C and 24°C, with a relative humidity range of 40–50%. The EG of subjects was exposed in the chamber continuously for 4 h/day for 4 days. The duration and severity of exposure have been validated and established in the previous studies.[7,16] The IHE was conducted in the morning; the subjects were seated in the chamber after breakfast.
6MWT
The 6MWT is a self-paced test measuring functional capacity. The 6MWT was performed outdoors along a hard surface course measuring approximately 30 m, as per standard guidelines (ATS 2002). The 6MWT was conducted once at sea level and on the 3rd day of each of the subsequent altitudes. 6MWT was conducted on both groups. Resting vital parameters (HR, SpO2, and BP) were measured after subjects had been seated for 5 min. SpO2 and HR were measured with a finger pulse oximeter (Model MU 300). Two investigators recorded the values after 30 s when the values stabilised. The BP was measured with a portable digital monitor (OMRON). The 30 m track was demarcated with cairns/flags at every 10 m. Subjects were instructed to walk as fast as they could without running. Every 60 s duration was communicated during the 6MWT. After the 6MWT was completed, vital parameters were repeated immediately. Two investigators observed the subjects and recorded their covered distance. Dyspnoea and fatigue were assessed using the modified Borg scale (0–10). At altitude, the subjects were asked to wear insulated gloves during 6MWT to ensure that the perfusion in the fingers was adequate.
Statistical analysis
T-test was used to compare means between EG and CG using SPSS 2.0, and Microsoft Excel was used for graphical representation. Association analysis was performed using OR analysis using SPSS 2.0.
RESULTS
Our findings reveals that at Stage I, the EG had improved SpO2 levels in both at rest EG (AR1), i.e. before 6MWT, and after 6MWT (E1) as compared to CG, i.e. at rest CG (AR2) and after 6MWT (C2). The HR, BP, and Borg’s scale were higher in EG, and EG had a better mean 6MWT (605 ± 52.79) as compared to CG and it is statistically different (P = 0.03) [Table 3]. At Stage II, EG continues to sustain its quality of SpO2 AR1 versus AR2 (P = 0.03) E1 versus C2 (P = 0.00). Furthermore, EG individuals had better HR and BP levels, albeit not significant. A significant difference in Borg’s scale between EG and CG was also observed at stage II, P < 0.00. At Stage II, the AR1 group had better HR (P = 0.00) as compared to AR2, and after 6MWT, the E1 group had better HR as compared to the C2 though not significant. There was a significant difference between E1 (146.38 systolic blood pressure [SBP]) and C2 (138.50 SBP), P = 0.00 (SBP) and diastolic blood pressure, P = 0.05. EG Groups had significantly improved Borg’s scale (0.77 vs. 1.58, P = 0.00), given that both the groups did not experience any physical stress exertion based on these Borg inspires results, EG was performing better as indicated by SpO2 in the context of breathlessness. Delta SpO2 had a suggestive P value as compared to the CG group (0.07). As evident from [Figure 1], individuals who went through IHE had improved Delta SpO2, with suggestive significance (P = 0.06) at Stage II as compared to the CG. At Stage I [Table 4], OR analysis showed no significant difference, but the IHE individual had a higher percentage of SpO2 (93.33%). In Stage II [Table 5], 83.34% of CG had lower SpO2. There was a significant difference between IHE and CG group and where CG was at significant risk for hypoxia (8.00 [1.21–52.60], P = 0.03).
Variables | EG | CG | AR1 versus AR2 | E1 versus C2 | ||
---|---|---|---|---|---|---|
At rest (±SD), AR1 | 6MWT (±SD), E1 | At Rest (±SD), AR2 | 6MWT (±SD), C2 | P-value | P-value | |
SpO2(%) | 93.47 (1.45) | 91.87 (1.87) | 92.60 (1.68) | 90.33 (2.96) | 0.14 | 0.09 |
HR (bpm) | 80.53 (12.07) | 94.80 (19.73) | 76.60 (13.28) | 85.40 (14) | 0.40 | 0.14 |
SBP (mmHg) | 131.13 (8.87) | 149 (17.79) | 130.80 (13.60) | 146.33 (15.22) | 0.93 | 0.66 |
DBP (mmHg) | 79.53 (9.64) | 93.33 (7.37) | 82.47 (8.6) | 93.27 (6.37) | 0.38 | 0.98 |
Borg’s scale | 0.70 (0.25) | 0.67 (0.41) | 0.81 | |||
6MWT (meter) | 605.33 (52.79) | 573.33 (13.63) | 0.03 | |||
Delta of SpO2(%) | 1.60 (1.63) | 2.27 (2.46) | 0.38 |
n=15 (EG) and n=15 (CG), AR1: At-rest experimental group, AR2: At-rest control group, E1: Experimental group after 6MWT, C2: Control group after 6MWT, EG: Experimental group, CG: Control group, 6MWT: 6-min walk test, SpO2: Oxygen saturation, HR: Heart rate, DBP: Diastolic blood pressure, SBP: Systolic blood pressure, SD: Standard deviation
Stage-I | EG (%) | CG (%) | χ2 | OR (CI-95%), P value |
---|---|---|---|---|
SpO2% -Normal | 14 (93.33) | 10 (66.67) | 0.17* | 7.0 (0.70–69.40), 0.09 |
Low | 1 (6.67) | 5 (3.33) |
Yates*, EG: Experimental group, CG: Control group, SpO2: Oxygen saturation, OR: Odds ratio, CI: Confidence interval
Stage-II | EG (%) | CG (%) | χ2 | OR (CI-95%), P value |
---|---|---|---|---|
SpO2% normal | 8 (61.54) | 2 (16.66) | ||
Low | 5 (38.46) | 10 (83.34) | 0.06* | 8.00 (1.21–52.60), 0.03 |
Yates*, EG: Experimental group, CG: Control group, SpO2: Oxygen saturation, OR: Odds ratio, CI: Confidence interval
DISCUSSION
Our finding illustrates the benefit of IHE in acclimatisation, as evident from the improved level of SpO2 in Stages I and II, respectively, amongst the EG. Studies have shown that IHE may improve oxygen-carrying capacity.[24,25] A recent study indicated that individual undergoing intermittent hypoxia protocol had significant improvement in their SpO2 levels.[26] Our findings are in concordance with these previous studies. The purpose of IHE in this context is to stimulate a hypoxic environment for the body at sea level to facilitate acclimatisation at HA. Hence, IHE plays a pivotal role in rapid acclimatisation and reducing AMS. To further validate the benefits of IHT, 6MWT was used as an assessment tool in the present study. This non-invasive tool has proven effective in assessing an individual’s health status,[27] with several studies confirming its utility in diverse areas.[28-30] The convenience and cost-effectiveness associated with 6MWT make it a valuable tool for assessing comorbidities.[31] 6MWT conducted at different stages showed that the EG had better performance than the CG, though not statistically significant. Albeit insignificant, the value of ΔSpO2 at Stage II between the EG (1[±1.08]) and the CG (2[±1.73]) indicates that EG individuals are better acclimatised to hypoxia in HA. Similar finding was observed in our pervious study where moderate altitude natives (2100 m) had better ΔSpO2 as compared to lowlander individuals (Sea level) at HA (4350 m).[32] [Table 6] demonstrates that the EG, had better ΔSpO2 (1), as compared to CG (ΔSpO2 2.08) and achieved a better 6MWT score (503.85 m +63.90) than CG (485.83 m +47.66). Hence, 6MWT, in this study, seems to be the most effective and efficient method to assess the benefit of IHE. While EG exhibited better SpO2 at Stage I, a significant difference emerged between E1 and C2 in SpO2 levels at Stage II, as well as between AR1 and AR2 groups. It is evident that IHE is beneficial for acclimatisation, and the findings are in concordance with Behrendt et al. 2022 systematic review, where the findings reveal that intermittent hypoxic training increased physical performance considerably.[33] At rest, there was no significant difference in SBP; however, after 6MWT, significant difference was observed amongst E1 and to C2, P = 0.00 [Table 6]. Regression analyses were performed on both groups at different stages of altitudes with SpO2 as a risk variable. At Stage I, no association was observed, but at Stage II, there was a significant difference between EG and CG where 83.3% of CG had SpO2 Levels <90% and were at increased significant risk for hypoxia (8.00 [1.21–52.60], P = 0.03). However, further studies are required on a higher number of sample sizes to support these current findings. Furthermore, ΔSpO2 affirms that EG’s SpO2 improves as compared to CG [Figure 1]. This significant difference in OR between EG and CG is evidence that IHE is improving the O2 delivery. The measurement of FiO2, PO2 and the ability of haemoglobin to carry saturated O2 is beyond the purview of the study, but the evidence is promising that EG had better 6MWT and significant improvement in SpO2 in HA as compared to CG. The limitation of the study is that several data could not be collected due to logistical complications in HA locations. In addition, the findings define the role of IHE as a non-invasive tool. Moreover, it also validates 6MWT as a significant tool for assessing IHE response. IHE is a promising non-pharmacological intervention strategy for improving peak oxygen consumption and exercise tolerance in the context of SpO2.
Variables | EG | CG | AR1versus AR2 | E1versus C2 | ||
---|---|---|---|---|---|---|
At rest (±SD), AR1 | 6MWT (±SD), E1 | At rest (±SD), AR2 | 6MWT (±SD), C2 | P-value | P-value | |
SpO2(%) | 90.23 (1.73) | 89.23 (1.7) | 88.75 (2.22) | 86.67 (2.3) | 0.03 | 0.00 |
HR (bpm) | 79.92 (9.17) | 87.23 (13.67) | 95 (14.12) | 95.08 (13.44) | 0.00 | 0.12 |
SBP (mmHg) | 127.31 (9.42) | 146.38 (16.23) | 127.25 (10.56) | 138.50 (12.06) | 0.98 | 0.00 |
DBP (mmHg) | 81.15 (8.40) | 88.08 (7.11) | 83.50 (9.11) | 81.51 (9.14) | 0.87 | 0.05 |
Borg’s scale | 0.77 (0.26) | 1.58 (0.51) | 0.00 | |||
6MWT (meter) | 503.85 (63.90) | 485.83 (47.66) | 0.43 | |||
Delta of SpO2(%) | 1 (1.08) | 2.08 (1.73) | 0.07 |
n=13 (EG) and n=12 (CG), AR1: At-rest experimental group, AR2: At-rest control group, E1: Experimental group after 6MWT, C2: Control group after 6MWT, EG: Experimental group, CG: Control group, 6MWT: 6-min walk test, SpO2: Oxygen saturation, HR: Heart rate, SD: Standard deviation
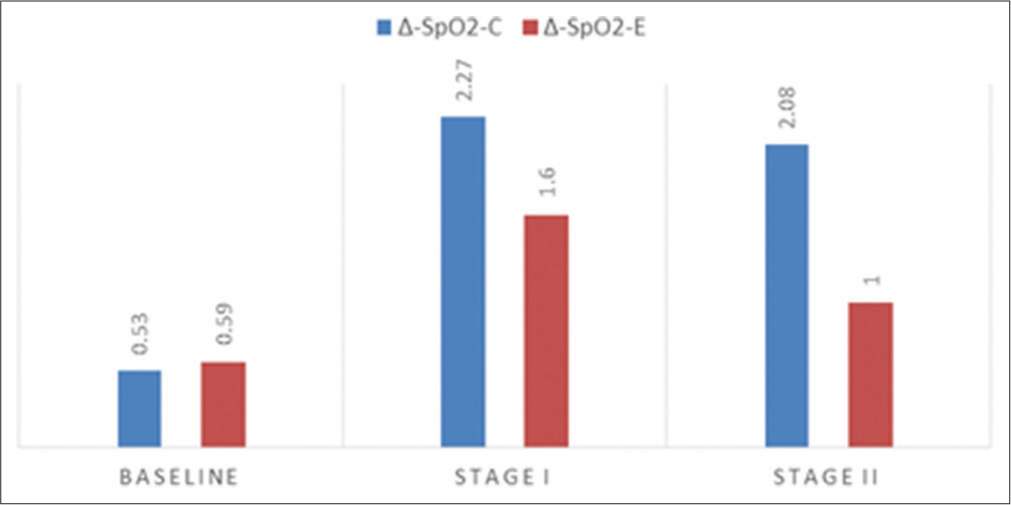
- Comparison of ΔSpO2-C (control group) and ΔSpO2-E (experimental group) value in different stages (Stage I = P value-0.36 and Stage II = 0.06). SpO2: Oxygen saturation.
CONCLUSION
The findings elucidate the benefits of IHE in rapid acclimatisation at HA and the role of 6MWT as a validating tool for its assessment. The SpO2 levels were significantly higher amongst IHE individuals. The risk of hypoxia amongst CG was significantly high with an OR of eight-fold. The finding is conclusive of the benefit of IHE.
Acknowledgement
The authors would like to thank the study participants who provided their valuable time. The authors would also like to thank the Director of DIPAS, DRDO, New Delhi for providing necessary support during the study.
Ethical approval
The study protocol was approved by the institute’s ethical committee No: IHEC/DIPAS/VI-C-01/2022 dated 8.6.2022.
Declaration of patient consent
Patient’s consent not required as there are no patients in this study.
Conflicts of interest
There are no conflicts of interest.
Use of artificial intelligence (AI)-assisted technology for manuscript preparation
The author(s) confirms that there was no use of artificial intelligence (AI)-assisted technology for assisting in the writing or editing of the manuscript and no images were manipulated using AI.
Financial support and sponsorship
Nil.
References
- High-altitude illness. Emerg Med Clin North Am. 2004;22:329-55, viii
- [CrossRef] [PubMed] [Google Scholar]
- High altitude medicine In: Auerbach PS, ed. Wilderness medicine (5th ed). Philadelphia, PA: Mosby Elsevier; 2007.
- [CrossRef] [Google Scholar]
- Mechanisms of action of acetazolamide in the prophylaxis and treatment of acute mountain sickness. J Appl Physiol (1985). 2007;102:1313-22.
- [CrossRef] [PubMed] [Google Scholar]
- Effect of intermittent normobaric hypoxia exposure on acclimatization to high altitude by air induction. Asian J Med Sci. 2021;12:59.
- [CrossRef] [Google Scholar]
- Intermittent hypoxia: An alternative to acclimatization to high altitude and enhancement of athletic performance? Am J Med Sports. 2002;4:385-91.
- [Google Scholar]
- Current trends in altitude training. Sports Med. 2001;31:249-65.
- [CrossRef] [PubMed] [Google Scholar]
- Hypoxic conditioning: A novel therapeutic solution for load-compromised individuals to achieve similar exercise benefits by doing less mechanical work! Br J Sports Med. 2020;17:944-5.
- [CrossRef] [PubMed] [Google Scholar]
- Hypoxic conditioning as a new therapeutic modality. Front Pediatr. 2015;3:58.
- [CrossRef] [PubMed] [Google Scholar]
- Pre-acclimatization to high altitude using exercise with normobaric hypoxic gas mixtures. Int J Sports Med. 1992;13(Suppl 1):S213-6.
- [CrossRef] [PubMed] [Google Scholar]
- Letter to the editor: Preacclimatization for expeditions to extreme altitude: An opinion position from the union Internationale des associations d'Alpinisme medical commission. High Alt Med Biol. 2020;21:303-4.
- [CrossRef] [PubMed] [Google Scholar]
- Use of a hypobaric chamber for pre-acclimatization before climbing Mount Everest. Int J Sports Med. 1992;13(Suppl 1):S216-20.
- [CrossRef] [PubMed] [Google Scholar]
- Rapid ascents of Mt Everest: Normobaric hypoxic preacclimatization. J Travel Med. 2020;27:taaa099.
- [CrossRef] [PubMed] [Google Scholar]
- Effect of intermittent normobaric hypoxia exposures on acute mountain sickness during acute ascent to 3500 m in Indian army personnel Def. Life Sci J. 2018;3:209-15.
- [CrossRef] [Google Scholar]
- Six-minute-walk test in idiopathic pulmonary fibrosis: Test validation and minimal clinically important difference. Am J Respir Crit Care Med. 2011;183:1231-7.
- [CrossRef] [PubMed] [Google Scholar]
- A qualitative systematic overview of the measurement properties of functional walk tests used in the cardiorespiratory domain. Chest. 2001;119:256-70.
- [CrossRef] [PubMed] [Google Scholar]
- Postexercise peripheral oxygen saturation after completion of the 6-minute walk test predicts successfully reaching the summit of Aconcagua. Wilderness Environ Med. 2010;21:309-17.
- [CrossRef] [PubMed] [Google Scholar]
- Pulse oximetry after 6-minute walk test and summit success on Kilimanjaro. Wilderness Environ Med. 2012;23:95-6.
- [CrossRef] [PubMed] [Google Scholar]
- The 2018 Lake Louise acute mountain sickness score. High Alt Med Biol. 2018;19:4-6.
- [CrossRef] [PubMed] [Google Scholar]
- Study of oxygen saturation by pulse oximetry and arterial blood gas in ICU patients: A descriptive cross-sectional study. JNMA J Nepal Med Assoc. 2020;58:789-93.
- [CrossRef] [PubMed] [Google Scholar]
- Reference values for oxygen saturation from sea level to the highest human habitation in the Andes in acclimatised persons. Thorax. 2018;73:776-8.
- [CrossRef] [PubMed] [Google Scholar]
- Enhanced cerebral perfusion during brief exposures to cyclic intermittent hypoxemia. J Appl Physiol. 2017;123:1689-97.
- [CrossRef] [PubMed] [Google Scholar]
- Intermittent hypoxia as a means to improve aerobic capacity in Type 2 diabetes. Med Hypotheses. 2017;100:59-63.
- [CrossRef] [PubMed] [Google Scholar]
- Short exposure to intermittent hypoxia increases erythropoietin levels in healthy individuals. J Appl Physiol (1985). 2021;130:1955-60.
- [CrossRef] [PubMed] [Google Scholar]
- Development technologies for the monitoring of six-minute walk test: A systematic review. Sensors (Basel). 2022;22:581.
- [CrossRef] [PubMed] [Google Scholar]
- Intermittent hypoxia increases exercise tolerance in patients at risk for or with mild COPD. Respir Physiol Neurobiol. 2009;165:97-103.
- [CrossRef] [PubMed] [Google Scholar]
- Preoperative change in 6-minute walk distance correlates with early weight loss after sleeve gastrectomy. JSLS. 2014;18:3.
- [CrossRef] [PubMed] [Google Scholar]
- Six-minute walk test: Clinical role, technique, coding, and reimbursement. Chest. 2020;157:603-11.
- [CrossRef] [PubMed] [Google Scholar]
- Study to find out the efficacy of 6-minute walk test in assessing response to medical intervention in chronic obstructive pulmonary disease patients. Int J Adv Med. 2016;3:538.
- [CrossRef] [Google Scholar]
- Oxygen saturation response to exercise VO2 at 2100 m and 4350 m in women mountaineering trainees. Indian J Physiol Pharmacol. 2003;47:43-51.
- [Google Scholar]
- Effects of intermittent hypoxia-hyperoxia on performance-and health-related outcomes in humans: A systematic review. Sports Med Open. 2022;8:70.
- [CrossRef] [PubMed] [Google Scholar]